ENVIRONMENTALLY SOUND
SMALL-SCALE WATER PROJECTS
GUIDELINES FOR PLANNING
BY
GUS TILLMAN
COORDINATION IN DEVELOPMENT
VOLUNTEERS IN TECHNICAL ASSISTANCE
[C] 1981 CODEL
Cover design and illustrations by Susann Foster Brown
TABLE OF CONTENTS
PREFACE
AUTHOR'S NOTE
1. WATER - USERS AND USES
2. WATER AND ENVIRONMENT
3. WATER AND HEALTH
4. ENHANCEMENT, DEVELOPMENT AND PROTECTION
5. SANITATION AND WASTE TREATMENT
6. WATER AND AGRICULTURE
7. AQUATIC PRODUCTS
8. WATER AND ENERGY
9. PLANNING
APPENDIX I. BIBLIOGRAPHY OF CITED REFERENCES
APPENDIX II. WHERE TO ORDER REFERENCES
PREFACE
This is the second volume of the Guidelines for Planning
series. The first volume, Environmentally Sound Small
Scale Agricultural Projects, was jointly published in 1979
by VITA and The Mohonk Trust. The remainder of the series
is being produced by CODEL and published jointly by VITA
and CODEL. The booklets can be ordered from VITA.
This booklet has been written for community development
workers in developing countries who are not technicians in
the area of water resources. It is meant to serve as a
general guide when planning environmentally sound
small-scale
water projects, that is, projects which protect and
conserve natural resources in a manner which allows
sustainable
development to take place. Sources of more
detailed and specific information are listed in the text.
CODEL is grateful to members of the CODEL Environment and
Development Committee who have supported this effort and
commented on the material:
Father John
Joe Braun, Missionaries of Africa,
Committee Chairperson
Ms. Elizabeth
Enloe, Church World Service
Mr. George
Gerardi, Attorney at Law
Mr. George
Mahaffey, The Peace Corps
Dr. Ragnar
Overby, The World Bank
Ms. Agnes
Pall, International Division, YMCA
Mr. C.
Anthony Pryor, Center for Integrative
Development
Mr. A. Keith
Smiley, Mohonk Consultations on the
Earth's
Ecosystem
Pastor Charles Fluegel, a former Committee member, deserves
special thanks for his thoughtful, practical contributions.
In addition, two other former members of the
Committee should be recognized for their involvement in
the project: Miss Marion Morey and Mr. Michael Hayes.
CODEL is pleased to publish a booklet written by Dr. Gus
Tillman whose environmental training, expertise and overseas
experience make him a unique resource to the development
community. Dr. Tillman also serves on the CODEL
Committee.
Special appreciation is reserved for Mr. Philip W. Quigg
for his invaluable editorial and technical expertise.
Several persons reviewed the draft manuscript: Dr. John
M. Kalbermatten, Mr. James H. Patric, Dr. Daniel A. Okun,
Mr. R. Paul Chakroff, Ms. Marilyn S. Chakroff and Dr.
Patricia Rosenfield. In addition, the book was reviewed
by VITA volunteers and AID personnel, among them Ms. Molly
Kux, AID Office of Forestry, Environment and Natural
Resources.
Ms. Kux and Mr. Albert Printz, AID Environmental
Coordinator,
have been a constant source of support for the Environment
and Development Program and especially the publication
series. We are also appreciative of the continuing
support of the AID Office of Private and Voluntary
Cooperation.
We welcome comments from readers of the book; a
questionnaire is enclosed for your convenience. Please
share your reactions with us.
Rev. Boyd Lowry, CODEL
Ms. Carol Roever, CODEL
Ms. Helen L. Vukasin, CODEL
ABOUT CODEL
Coordination in Development (CODEL) is a private, not-for-profit
consortium of 38 development agencies working in
developing countries. CODEL funds community development
activities which are locally initiated and ecumenically
implemented. These activities include health, agricultural
and career training projects, among others.
The Environment and Development Program of CODEL serves
the private and voluntary development community by providing
workshops, information and materials designed to document
the urgency, feasibility and potential of an approach
to small-scale development which stresses the
interdependence
of human and natural resources. This booklet is one
of several materials developed under the Program to assist
development workers in taking the physical environment
into account during project planning, implementation and
evaluation. For more information, contact CODEL at 475
Riverside Dr., Room 1842, New York, NY 10115 USA.
ABOUT VITA
Volunteers in Technical Assistance (VITA) is a private
nonprofit international development organization. It
makes available to individuals and groups in developing
countries a variety of information and technical resources
aimed at fostering self-sufficiency--needs assessment and
program development support; by-mail and on-site consulting
services; information systems training. VITA promotes
the use of appropriate small-scale technologies, especially
in the area of renewable energy. VITA's extensive
documentation center and worldwide roster of volunteer
technical experts enable it to respond to thousands of
technical inquiries each year. It also publishes a quarterly
newsletter and a variety of technical manuals and
bulletins. For more information, contact VITA at 1815
North Lynn Street, Suite 200, Arlington, VA 2209 USA.
Published with support from the United States Agency
for International Development, Grant AID/SOD/PDC-G-0235
AUTHOR'S NOTE
Users, Uses and Mea Culpa
This manual is written for all those who are planning,
implementing, or responsible for a water project to benefit
small segments of the world's urban or rural poor.
Depending on the reader's level of expertise, the manual
may be criticized for over-simplification of complex
topics or for being too technical. It is meant to provide
a beginning for ecological analysis and a reference to the
necessary technical materials. The manual is best suited
as an initial guide for planning and discussion with
community
leaders.
In using this manual, it is hoped that the development
worker will read the section which applies to the proposed
project and then select one or more technical references
from the bibliography. The worker may then write to the
distributors (Appendix II) to obtain a copy of the relevant
references.
The author acknowledges with thanks the fine editorial
work of Philip Quigg as well as assistance from Julie
Morgan and typist Phyllis Haight of the Cary Arboretum.
References listed in Appendix I were invaluable. The
author accepts all responsibility for technical sins of
commission and omission.
G.T., Millbrook, New York
1981
1. WATER -
USERS AND USES <see image>
esw1x1.gif (437x437)
"The greatest problem in
communication is the
illusion that it has been
achieved."
Anonymous
Our planet contains an estimated 336 million cubic miles
of water. However, nearly 95% of this prodigious supply
is saltwater contained in the oceans and seas which cover
two-thirds of the earth's surface. Of the 5% that is
freshwater, all but 1% is frozen in polar ice caps or vast
northern glaciers. The remaining 3.36 million cubic
miles, which is at least theoretically available for human
use, is distributed approximately as follows:
Ground water
98.55%
Lakes
1.0
Soil (between particles)
0.2
Rivers and streams
0.1
Atmospheric vapor
0.1
Biological (in plant and
animal tissue)
0.05
If freshwater supplies were uncontaminated and equally
distributed around the globe, there would be little need
for water development projects and even less need for this
development manual. However, common sense and numerous
global studies tell us that water supplies are neither
uncontaminated, nor equally distributed; therein lies the
need for water development projects. According to a recent
global survey of 91 countries conducted by the World
Health Organization (WHO), 86% of the rural populations
(1.11 thousand million people) are without "reasonable
access to safe water." By region, the numbers and
percentages
of rural people without reasonable access to safe
water are as follows:
Africa
136.0 million 89%
Americas
92.1 million
76%
Eastern
Mediterranean 139.5 million
82%
Europe
23.3 million
56%
Southeast
Asia 661.7 million
91%
Western Pacific
59.0 million
79%
All regions
1,111.6 million
86%
(after Feachem, 1977)
In view of these staggering statistics, the World Health
Assembly set a seemingly modest target at the start of the
last decade: to give 25% of the rural populations of
developing nations reasonable access to safe water by
1980. However, just to maintain 1971 levels, world
population
growth would require new water supplies for 297
million additional people since the start of the decade.
In spite of the intensive and costly efforts to increase
safe water supplies, it is likely that a larger percentage
of rural people lack access to safe water in 1980 than in
1971. To gain ground, water development efforts will have
to increase in quantity and improve in quality. Therein
lies the principal need and purpose of this manual.
It is not always possible to obtain the professional
studies and analyses that might be desired. New
water-related
projects are increasingly being developed to meet
pressing local needs in agriculture and health without
benefit of professional scientific or engineering advice.
Small-scale projects are initiated, planned and often
implemented by highly motivated, experienced development
workers, who have insufficient technical skills and
experience
in water resource development. Given ideal conditions,
field workers can develop the necessary expertise
to plan and implement small-scale water projects. But
realistically, assignments are short-term, needs are at
crisis point, available experts are overworked on
large-scale
projects and literature is so difficult to obtain it
might as well be on the moon.
To address this reality, this manual was prepared as a
guide or aid in planning and executing environmentally
sound small-scale water resource projects. It is not
intended to replace technical literature or professional
advice when available, but to serve as a helpful substitute
when those sources of information are unobtainable or
as a guide to topics on which further information may be
needed. Through a discussion of the environmental factors
which relate to water development and use, the manual
encourages the incorporation of environmental considerations
into water development planning to increase the
probability of long-term project sustainability.
WHO SHOULD USE THIS MANUAL?
Anyone who must plan, review, supervise or implement
small-scale water resource projects and:
*
has limited experience in water resource
technology
or minimal
access to technical experts
*
wishes to learn more about the environmental
relationships
that affect
water resource projects
*
must prepare or review a report on the
environmental
aspects of
water development projects
*
must prepare a training program on
small-scale
water
development projects.
WHAT DOES THE MANUAL PROVIDE?
*
Basic ecological principles which relate to
water
resource
development
*
A guide for planning small-scale water
projects
*
Suggestions for low-cost techniques to avoid
adverse impacts
of water development
*
Basic information and resources for planning
and
implementing
projects in water supply, water conservation,
water distribution
systems, waste-water
treatment,
agriculture, energy and public health.
ECOLOGY AND THE ENVIRONMENT
Today the terms ecology and environment often are used
interchangeably, but they are not the same. Quite simply,
ecology is the study of the relationships and interactions
of the living and non-living parts of our surroundings.
The living parts include plants, animals and one-celled
organisms (e.g., bacteria and algae), while weather, soil,
rocks, energy, topography and water are some of the
nonliving
elements in our surroundings. All these factors
are interrelated, mostly in ways which we do not fully
understand, although many of the major relationships have
been defined by ecologists. We have learned that it is
impossible to alter one part of our surroundings without
producing changes in some other components. Since a water
development project will alter major parts of a particular
ecologic system, we know that other parts will change.
Through sound planning and implementation, we can try to
ensure that changes will not produce severe negative
effects.
Environment is an even simpler term to define because it
can be used interchangeably with surroundings, if one
keeps in mind that surroundings are all-inclusive, involving
all living and non-living parts. Within this definition,
it is proper to include man and the social and
cultural activities associated with humans. It is clear
from the definitions that ecology is the study of
environment;
therefore these are not interchangeable terms and
will not be used in this manual as though they were.
The natural environment is a term used to describe systems
which have evolved over millions of years, approaching a
harmonious, or perhaps, dynamic balance. In natural
systems, water cycles, soil fertility and plant-animal
relationships tend to be stable and predictable, although
often upset by natural catastrophes such as earthquakes,
floods, volcanic eruptions or lightning-induced fires.
Man, who has the ability to alter significant parts of a
system, produces artificial environments such as cities,
farms and lakes. The new environments contain many benefits
to be sure, but also may create many unfavorable
conditions: increased disease, contaminated water supplies,
deforestation, desertification and eroded soils.
Recently, through ecology, we have learned that if
artificial
environments can be made to function more like natural
environments, they tend to be more stable and predictable.
Therefore, it is in our best interest to understand
the functioning of a natural system so that the conditions
ensuring stability and long-lasting benefits can be
preserved,
at least in part, in man-devised projects. Scientists
often identify smaller units within the natural
system called ecosystems. See section on "Ecosystems"
in
Chapter 2.
2. WATER
AND ENVIRONMENT <see image>
esw2x7.gif (393x437)
". . .For in the wilderness
shall waters break out and
streams in the desert."
The Bible
The primary goal of water resource development is to
provide safe and reliable water supplies for human use.
This may involve the development of a new water source,
expansion of an existing source, improvements in collection
and delivery, or methods of water conservation. In
some cases, water quantity may be adequate, but inferior
quality requires a plan for purification or improved
sanitation.
In addition to developing potable water supplies
for human consumption and hygiene, communities often want
more water for agriculture or manufacturing to increase
employment opportunities and improve the standard of
living.
Even where water development projects are implemented with
the best intentions, unforeseen environmental factors can
produce negative effects, often outweighing the benefits
of the project. Dramatic increases in water-related
disease, loss of soil fertility, increased erosion and
changes in the hydrologic balance are some of the adverse
side effects of poorly planned water projects. Determining
the possible positive and negative effects may be the
most important task faced by planners of small-scale water
projects. Through the selection of an alternate technique
or a minor modification of a proposed project, many of the
unwanted consequences can be either reduced or avoided
altogether. An understanding of basic ecological concepts
and an awareness of environmental relationships can help
planners to judge the direction and magnitude of
environmental
changes that various alternatives might cause and
to assess the positive and negative effects of the possible
options.
It is in tropical regions, where most of the developing
countries are located, that we find the greatest inequities
in water distribution. The extensive arid and semiarid
regions of the tropics are plagued not only by inadequate
rainfall for crops, but also are subject to extreme
variations of rainfall from season to season and from year
to year. When rainfall is normal, it is often restricted
to brief intervals during the year--not long enough for
plant growing seasons. In the past, such lands were
sparsely occupied, usually by nomadic tribesmen who moved
their families and livestock from place to place to find
seasonal water and vegetation. Rising populations of
people and domestic animals have put intense pressure on
these small, seasonal sources, often resulting in their
destruction through overuse.
On the other hand, moist tropical areas receive amounts of
rainfall far in excess of that required for plant growth.
The excess water moves over or through the soil to form
immense tropical rivers such as the Amazon, Niger, Congo,
Mekong and Nile. The enormity of these river systems
illustrates the unequal distribution of freshwater: one
river, the Amazon, discharges approximately 20% of the
world's runoff of freshwater. This overabundance of water
can create serious problems, as we will see, if the natural
vegetation is altered for development purposes.
In both arid and moist regions, increased human activities
have produced changes in natural water cycles, some yielding
obvious agricultural or industrial benefits while
others have caused serious unforeseen consequences and
increased human suffering.
MAJOR SOURCES OF WATER FOR DEVELOPMENT PROJECTS
*
Precipitation, in the form of rain or snow,
cannot
yet be
effectively controlled by man. Water resource
projects must
be designed to accommodate the
wide range of
annual as well as seasonal precipitation.
*
Surface waters are the most obvious source
of
freshwater
that can be tapped. Lakes, ponds,
rivers,
streams, reservoirs and catchments are
examples of
surface water sources.
*
Ground water refers to the subsurface
supplies fed
by
precipitation and surface water. It is a major
reservoir for
freshwater. Ground water supplies
contained in
aquifers are relatively stable unless
influenced by
man's activities. When ground water
is within 3
meters of the land surface, it may move
upwards,
against gravity, through small soil pores
by a process
known as capillary action. It thus
becomes
available to shallow rooted plants, such as
most
agricultural crops. In some areas, or during
periods of
excessive rainfall, ground water may be
at or near the
surface, flooding the root zone and
killing most
plants. Ground water may also be held
in pockets far
down in the earth's crust and can be
reached only
by drilling deep wells through rock.
A shallow pocket
is also an aquifer. Some aquifers,
such as those
found in northern Africa, often
contain huge
volumes of water but were covered in
an earlier
geological time with impervious rock
layers which
do not permit recharge from surface
sources. These
"fossil water" aquifers have remained
in place for
thousands of years and since
they cannot be
replenished by natural means, they
must be
considered non-renewable resources.
The upper
level of the ground water is called the
water table
and how far it is beneath the surface
of the land is
as important to consider in water
development
projects as the volume of ground water
itself. The
height of the water table will change
with the seasons and the amount of
rainfall. The
relationships
of water table, ground water, surface
water,
geologic features and precipitation are
shown in
Figure 1.
esw1x11.gif (486x486)
*
Distillation and condensation are minor and
expensive
sources of
freshwater. Distillation and condensation
of a saline
water supply will produce
freshwater,
but require a large amount of energy.
Some small,
solar-powered stills can provide supplemental
water for
domestic use with inexpensive
materials.
Natural condensation of water vapor
(dew) is a
minor source of water for plants, but
has little or
no potential for human use.
THE HYDROLOGIC CYCLE
The movement of water from earth surfaces to the atmosphere
and back to the earth is called the hydrologic
cycle. It is the basis for all water development projects,
large and small, and a firm understanding of the
basic process and its vagaries are important to water
resource planning.
The hydrologic cycle involves evaporation, transpiration
(emission of water vapor from the leaves of plants),
condensation,
cloud formation, precipitation, surface runoff,
water storage and percolation (Figure 2). These processes
esw2x12.gif (540x540)
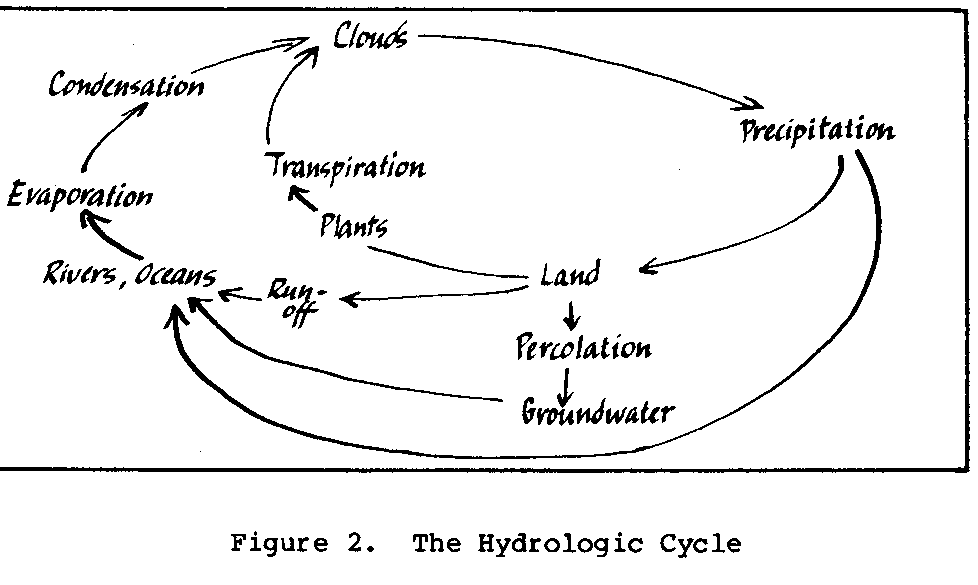
in turn affect the patterns, practices, quantity and
quality of human life. Most water development projects
seek to make minor local changes in the natural cycle so
as to provide additional human benefits in the form of
additional water supplies or water movement. Alteration
of the hydrologic cycle does not involve changes in the
basic processes, but rather in their rates or volumes.
When sunlight strikes a water surface, whether it be an
ocean, a river or saturated land, the water molecules get
warmer and begin to move faster. As the movement increases,
some molecules break away from the liquid surface
and move into the atmosphere as a gas, in this case, water
vapor. This process is known as evaporation and is
responsible
for most of the water vapor in the atmosphere.
Most of the global water evaporation takes place over the
oceans which comprise nearly 70% of the surface area of
the earth. When water and other liquids are evaporated
from land or water surfaces, the larger and slower
molecules,
such as salts and metals, are left behind.
Transpiration is the second major contributor of atmospheric
water vapor and it too is powered by energy from sunlight.
Water absorbed by plant root systems is passed
internally to the upper portions of the plant. In the
green portions of the plant, principally the leaves, some
water is used for photosynthesis, but most of the water is
passed through small pores or openings in the leaves. At
the leaf surface, the sunlight causes the water to change
to a vapor which then rises into the atmosphere as it is
heated. The amount of water moved by transpiration is
amazing; for instance, one hectare of corn can transpire
37,850 liters (10,000 gal.) per day or 1,900,000 liters
(500,000 gal.) per growing season. A single mature tree
may transpire up to 378 liters (100 gal.) per day. In
areas where soil moisture is excessive, plants are often
used, along with artificial drains, to remove the excess
moisture in the soil.
In analyzing moisture loss or water requirements for
agriculture,
evaporation and transpiration figures are combined
as evapotranspiration losses. Since both processes
are directly affected by temperature, relative humidity,
wind and available water, the rate of evapotranspiration
loss is an important indicator of the climate of the
area. More commonly, the climatic factors can be used to
estimate the evapotranspiration loss for proposed
agricultural
projects.
Condensation of water vapor in the atmosphere produces the
various cloud formations that will eventually lead to
precipitation.
The vapor gradually cools as it rises, and
eventually its molecules strike small particles, such as
dust, in the atmosphere and condense to form clouds. The
moisture laden clouds may cool further by passing over a
mountain range or by meeting a cold air mass. The sudden
cooling further compacts the water molecules into droplets
of water that fall as rain or in frozen form such as snow
or sleet. Topography, cloud altitude and prevailing wind
direction all contribute to the uneven distribution of
precipitation. Some areas, such as the windward slopes of
coastal mountains, may receive over 3000 mm per year while
the other side of the same mountains may not record any
precipitation for an entire year.
Most soils, especially if covered with vegetation, absorb
water and only the excess becomes part of the surface
runoff.
But if all or parts of the soil are even slightly
impervious to water due to rock cover, soil compaction or
baking, runoff will be heavy.
Surface water will either flow to the oceans, seep into
the ground water, or evaporate. Because surface water is
so precious in most countries, it may be used for several
human activities before it reaches the ocean. For instance,
surface water could pass through a hydroelectric
generator, flow on to an irrigated field, pass through an
industrial cooling system or be used for sewage removal
for a riverside city. Each use would have effects on
water quality and minor effects on parts of the hydrologic
cycle by increasing or decreasing the rate of evaporation
or percolation.
Rapid or uncontrolled surface runoff can produce flooding,
land slides and serious erosion. Even on a lesser scale,
runoff can have deleterious environmental effects. As it
moves across the land, the water picks up soil particles
and organic materials important to soil fertility. Their
removal from agricultural land decreases productivity. Of
course, if the particles of topsoil are deposited on
downstream
agricultural areas, those lands are improved. However,
the soil particles are usually transported to water
courses and are carried as suspended solids until deposited
as sediments in slow-moving bodies of water, such as
reservoirs, lakes or oceans. Although this may enrich
aquatic systems, it represents a serious loss to
agriculture.
Water falling on vegetation is more likely to remain in
place than water which strikes bare soil. The plant material
not only dissipates the force of the rainwater but
also impedes surface flow. Vegetation allows more time
for the soil to absorb the rainwater and also improves the
soil structure so that it can absorb more water at a
faster rate.
Once absorbed into the soil, the water can percolate down
to aquifers in rock or gravel, or strike an impervious
rock layer and move horizontally as a subsurface or ground
water flow. Some of the subsurface water will be taken up
by plants. The rest, if untapped by wells, will either
remain as ground water or eventually feed into streams or
rivers, becoming a part of the surface water system.
Since subsurface flows are more leisurely than surface
flows, the water remains available for human use for
longer periods.
In spite of the seemingly incredible amount of plants and
animals in the world, only a miniscule fraction of the
total freshwater supply is held in plant and animal tissue
and can be disregarded in planning for water resource
development.
This oversimplified explanation of the hydrologic cycle
indicates major areas for analysis before implementing a
water development project. It suggests where water is
likely to be found and is the basis for determining the
best methods to develop and protect the source.
ECOLOGICAL CONCEPTS IMPORTANT FOR WATER RESOURCE
DEVELOPMENT
Development implies change for the better--a change from
existing conditions or resource use to a system that is
safer or more productive; in other words, producing more
benefits for a larger number of people. Development
involves the alteration of resources or imposes a different
strategy for using the resources available. By
understanding ecological principles, developers can increase
the probability of success, i.e., there will be
more positive than negative effects.
Ecosystems
Since a study of ecological systems can be so all
encompassing,
the shorthand term "ecosystem" was coined to
define the smaller units which ecologists choose to
study. Thus, a scientist may study a forest of several
thousand hectares or a decaying log and yet investigate
principles and concepts common to both systems. The term
allows the scientist to place recognizable boundaries on
the area of investigation to fit the needs of research
objectives.
As with many scientific terms, "ecosystem" has
been widely
and often used erroneously and now has a much broader
meaning. Generally, the term applies to an area of
homogeneity
of one particular part of the natural system, such
as type of vegetation, amount of rainfall, topography or
physical feature. Further, ecosystems can be classified
as natural or artificial. In natural ecosystems, man is
not the dominant factor and changes tend to be minor
unless some catastrophic event occurs. In studies of
natural ecosystems, scientists have found dynamic balances
achieved through constant minor adjustments within the
system. These balances ensure relative stability in plant
and animal populations, minor fluctuations in water
movement,
and nutrient inputs nearly equal to nutrient losses.
If more had been known about the reasons for stability
and productivity of the natural ecosystems, the artificial
ecosystems created by man might have been a lot
more productive.
The biological bases of any ecosystem consist of three
major groups: producers, consumers and decomposers. The
ecosystem producers are the green plants, whether algae,
grasses, trees or weeds. By the process of photosynthesis,
plants combine carbon dioxide and water in the presence
of sunlight (for energy) to produce carbohydrates
(sugars and starches) and release oxygen as a by-product.
Using the carbohydrates for energy, the plants can take
other nutrients from the environment to produce fatty
acids, protein and vitamins, forming the energy and
nutritional
base for the other biological parts of the ecosystem.
The goal of many water development projects is to
supply water essential to these producers.
The consumers in the ecosystem are animals, including man,
which eat plants or other animals. In order to produce
energy, consumers must combine food with oxygen, releasing
small amounts of carbon dioxide and water in the process.
Animals use the energy for heat, growth, movement, and the
production of more complex chemical compounds, such as
fats and proteins, which are important in storing energy,
building new tissue or transmitting genetic materials to
offspring. Consumers require additional supplies of water
to construct new tissue to transport various chemical
compounds within the body, and to regulate internal
temperature.
Plants cannot absorb nutrients unless they are in very
simple chemical forms. For instance, the roots of a
forest tree cannot use the nutrients in a dead animal's
tissue unless the complex carbohydrates, fats and proteins
are broken down into simpler molecules which contain
carbon, nitrogen, potassium, calcium and phosphate.
Decomposers, mainly bacteria and fungi, break down the
large molecules in dead plant and animal tissues or wastes
into the simpler forms that can be used by plants. These
microscopic organisms play a vital role in the ecosystem,
for without their presence, the producers would not be
able to construct new tissues. Water development projects,
whether for agriculture or sanitation, must rely on
viable populations of decomposers to continue the recycling
of nutrients through the ecosystem.
The role of plants and animals in an ecosystem is not
limited to production, consumption and decomposition.
There are many other functions such as soil protection,
water retention, microclimate modification, pollination
and seed dispersal, just to name a few. So when someone
wishes to alter a part of an ecosystem to make it more
productive (or less hostile) for man, it is necessary to
examine aspects other than productivity.
WHAT HAPPENS WHEN NATURAL SYSTEMS ARE ALTERED?
The dominant natural feature of most land ecosystems is
the plant cover or vegetation. The type and density of
the vegetation influences soil structure and content,
water movement, nutrient balance, type and abundance of
animal populations and microclimate. When the native
plant cover is removed or changed for development, each of
these areas can be affected. For instance:
*
If vegetation is removed, wind can blow away
soil
particles and
organic matter in the topsoil, thereby
removing the
most fertile part of the soil.
*
The combined action of wind and sun on
exposed soil
increases
evaporation, often causing the soil to
become dry and
brittle, less likely to be suitable
for
agriculture.
*
Soil particles with attached nutrients, as
we have
noted, may be
dislodged by hard rainfall striking
the ground
rather than plant leaves. Runoff carries
the soil
particles and nutrients from the
land, where
they are needed to maintain soil fertility,
to waterways
where they are not needed. As
silt and
sediments, they may clog water courses,
kill fish,
increase flooding and fill reservoirs.
*
Nutrients carried into the waterways may
increase
the growth of
algae or waterweeds, rendering the
water unfit
for desirable animals, but more suitable
for animals
that transmit diseases (vectors).
The nutrients may also make the surface water
less
desirable for
human consumption.
*
Protection from flooding is reduced when
covering
vegetation is
removed. Plants maintain soil porosity
which helps
the soil absorb water. In addition,
the roots and
stems retard the flow of surface
water. With
the vegetation removed, water
does not
percolate easily into the soil if the
terrain is not
level, but tends to run over the
surface in
sheets or rivulets, increasing soil
erosion and
the danger of flooding in downstream
areas.
*
Traditional sources of wood, fruits,
medicines and
cooking herbs
may be lost if the native vegetation
is removed or
replaced by agricultural crops.
*
Diversity of animal life is decreased.
Animals
which depend
upon natural plants for food or shelter
will be forced
to move if the vegetation is
removed by
man. These animals are often replaced
by animals
that live all too comfortably with
domestic crops
or livestock--basically agricultural
pests. The
animal population may decrease the
number of
different species, but increase the
number of
individuals, which may be the unwanted
types.
There are, of course, many other effects on the environment
when natural ecosystems are altered; it would be
impossible to make a complete list. Some changes are complex
and difficult to predict; others are rather straightforward.
In water resource planning, we obviously want to
capitalize on the changes which are beneficial and minimize
those changes which are detrimental. Each water
development project will cause changes (environmental
impacts) and proper planning involves an analysis of these
impacts before the project is implemented. The following
chapters should help development workers plan and implement
water resource projects within an environmental
framework that identifies impacts of alternative schemes
and helps to ensure the selection of the most appropriate
option.
3.
WATER AND HEALTH <see image>
esw3x21.gif (353x353)
"Where water goes, disease
follows in its wake."
Anonymous
Small-scale water development projects are intended to
improve the quality of the human environment. Most
health-related projects are designed to provide potable
water, safe excreta disposal, or water for agriculture to
improve nutrition. If well-planned and well-designed, the
projects are successful and the people benefit. However,
if planning is haphazard and designs are incomplete, there
is a strong possibility that disease may increase. In
many cases, a water project may bring about a decrease in
one type of disease but cause an increase in a more severe
type. This unfortunate outcome is most common in projects
designed to improve local agriculture or to provide
additional
energy through the use of water.
POSSIBLE EFFECTS OF WATER DEVELOPMENT PROJECTS ON HUMAN
HEALTH
*
Water carries microscopic organisms that can
cause
disease in
humans and livestock. The microscopic
organisms
include bacteria, viruses, fungi and
single-celled
protozoans.
*
Water provides an environment necessary for
the
development of
many animals (e.g., snails and
insects) that
transmit diseases. These animals,
called
vectors, rarely cause sickness directly but
instead carry
the microscopic organisms that cause
serious
illness. Flies and mosquitoes, especially,
help to pass germs from sick people to
healthy
people,
thereby spreading the disease. In many
cases, the
insect spends only a part of its life or
life cycle in
water, but can transmit disease to
humans without
their direct contact with infected
water
supplies.
*
Water sources can provide suitable
environments for
animals that
are extremely common parasites of
humans. These
parasites, which can be single-celled
organisms,
flat worms or round worms, are
responsible
for the world's most common diseases,
causing
massive expenditures for medical care and
treatment as
well as terrible suffering. Schistosomiasis,
filariasis and
amoebic dysentery are
common examples
of parasitic diseases. Table 1
lists some of
the major water-related diseases.
*
Water from polluting industries or
agriculture can
carry toxic
chemicals that cause grave illnesses or
death if the
water is used for human consumption.
*
Health is affected by the amount of water
available
for personal
hygiene: washing clothes, bathing and
washing of
utensils and household items. If supplies
are limited,
personal hygiene is often neglected.
A safe supply
of water for personal use is
essential to
good health.
*
Water is directly and indirectly related to
proper
nutrition.
Each adult must have a water intake of
at least 6
liters per day to maintain adequate body
fluids. In
addition, agricultural produce requires
water for
survival and growth. If water supplies
are not
sufficient, agricultural production will
decline and
adversely affect nutrition.
TABLE 1
WATER AND HEALTH
Disease
Infectious Agent
Role of Water
Schistosomiasis
Helminth worm
Direct Transmission/
(Bilharziasis)
skin penetration
Diarrhea/enteritis
Symptom of many
Direct Transmission/
agents
ingestion
Hepatitis (Infectious)
Virus
Direct Transmission/
ingestion
Cholera
Bacteria
Direct Transmission/
ingestion
Typhoid Fever
Bacteria
Direct Transmission/
ingestion
Ascariasis
Helminth worm
Direct Transmission/
ingestion
Dracontiasis
Helminth worm
Direct Transmission/
ingestion
Amoebic Dysentery
Protozoa
Occasional Transmission
Bacillary Dysentery
Bacteria
Occasional
ingestion
Malaria
Mosquito
Vector habitat
Filariasis
Mosquito
Vector habitat
Onchocerciasis
Black Fly
Vector habitat
Yellow Fever
Mosquito
Vector habitat
Flukes
Helminth worm
Habitat of
intermediate host
COMMON WATER-RELATED DISEASE AND CONTROL
Of the diseases listed in Table 1, four specific diseases
and one general category of disease are especially
significant
in terms of their total incidence, their widespread
distribution and their long-term impacts on human
populations
as shown below.
TABLE 2
ESTIMATED WORLD-WIDE PREVALENCE OF CERTAIN
DISEASES RELATED TO WATER RESOURCE DEVELOPMENT
Disease
Prevalence
Schistosomiasis (Bilharziasis)
200,000,000
Filariasis
200,000,000
Onchocerciasis
40,000,000
Malaria
25,000,000
Enteric
Disease Unknown
(after McJunkin,
1975)
Schistosomiasis
This disease, also known as Bilharziasis, is caused by
worms which spend a part of their lives in snails before
infecting humans. There are three species of the
schistosomes
which cause disease throughout the tropical and subtropical
parts of the globe. Schistosoma mansoni is found
in central and southwest Africa and is increasing in
eastern and northern South America. S. haematobium <see
figure 4> is
esw4x26.gif (486x600)

found throughout Africa, in many areas overlapping with
S. mansoni. <see figure 3> S. japonicum <see figure
4> is
esw3x26.gif (486x600)

restricted to parts of Asia.
Like most intestinal worms (helminths) schistosomes
require two different hosts during their lifetime. The
adult worms, 7 to 26 mm long, live either in the intestines
(S. mansoni and S. japonicum) <see figures 3 & 4>
or in the bladder
(S. haematobium) of man or other animals. After mating, the
female lays eggs that are passed out of the human body in
feces or urine. To survive, the eggs must reach water
within a month and, if successful, the eggs hatch into
small swimming larvae called miracidia. These larvae must
penetrate the skin of certain species of freshwater snails
within 24 hours or die. Once inside the snail, each
miracidium
reproduces asexually, producing thousands of larvae
called cercariae. These forked tailed larvae leave the
snail and swim about vigorously, searching for a suitable
host. Man is the principal host for S. mansoni and S.
haematobium, although infections of S. mansoni have been
reported in baboons, dogs, cattle, rodents and other small
mammals. In S. japonicum, the non-human hosts play a more
important role. If the cercariae do not find a host within
72 hours, they perish; but if they make contact with
human skin, they quickly penetrate and enter the blood
stream of their new host. After reaching the liver via
the blood vessels, the larvae mature into adult worms;
they mate and migrate to veins in the intestinal or
urinary tract where they may live for years, constantly
producing new eggs. (Figure 5.)
esw5x27.gif (486x486)
The symptoms of schistosomiasis caused by S. mansoni and
S. japonicum are very similar. After infection, a skin
rash may appear, diarrhea is common and the liver may
enlarge. The diarrhea continues and the abdomen enlarges
and is painful when the adult worms begin to lay eggs.
In S. haematobium, the bladder becomes infected, producing
internal lesions which usually result in bloody urine.
Diarrhea or dysentery is not common with S. haematobium.
Medicines for treating schistosomiasis are expensive and
often cause undesirable side effects. The disease is more
debilitating than deadly, although severe infection can
cause death. The weakened physical condition of the
victim also increases the susceptibility to other
diseases.
Filariasis
The parasitic roundworms (nematodes) which cause filariasis
also require two hosts. The adult worms live and reproduce
within human lymphatic tissue, a part of the circulatory
system. The female worm produces smaller worms
called microfilariae which may be ingested by blood-sucking
mosquitoes. In the mosquito the larvae undergo
changes until they reach an infective stage. As the
mosquitoes
feed on another human, the larvae pass into the
host's circulatory system where they move to lymphatic
tissue and there develop to maturity.
The symptoms of filariasis consist of painful swellings of
the lymphatic glands under the arm and especially of the
groin, genitals and thighs. If the infection is extreme,
grotesque enlargements of the breasts, genitals or lower
extremities may occur. This condition, called elephantiasis,
is severely debilitating, as well as disfiguring.
The role of water in the spread of filariasis is to provide
breeding habitat for the many types of mosquitoes
which are capable of transmitting the disease. Unlike the
conditions for schistosomiasis, direct human contact with
water is not necessary. Because so many kinds of mosquitoes
carry the disease, it is widespread in all tropical
regions (Figure 6). As with schistosomiasis, the disease
esw6x29.gif (600x600)

does not respond well to medical treatment except in minor
infections.
Onchocerciasis
This disease, commonly called River Blindness, is caused
by another nematode, transmitted by the bite of a black
fly. It is most common in Africa (Figure 7), although it
esw7x31.gif (600x600)

is found in parts of Central and South America. The adult
worms live just beneath the skin of humans, where the
female produces microfilariae. When a certain species of
black fly (Simulium sp.) bites an infected human, some of
the microfilariae go into the fly where they develop into
infective larvae. As the fly bites another human, the
larvae enter the blood vessels in the skin to complete the
life cycle.
At first the worms produce a severe itching of the skin;
later, thickening and loss of pigmentation in the infected
areas are common symptoms. The severity of the disease
increases when the worms reach the eye, where they can and
often do cause blindness. In some areas, more than 10% of
the population may be infected by this terrible disease
which does not respond well to present-day medicines.
Malaria
The parasite responsible for this widespread tropical
disease is a one-celled organism (protozoan) from the
genus Plasmodium (Figure 8). Four different species of
esw8x32.gif (600x600)
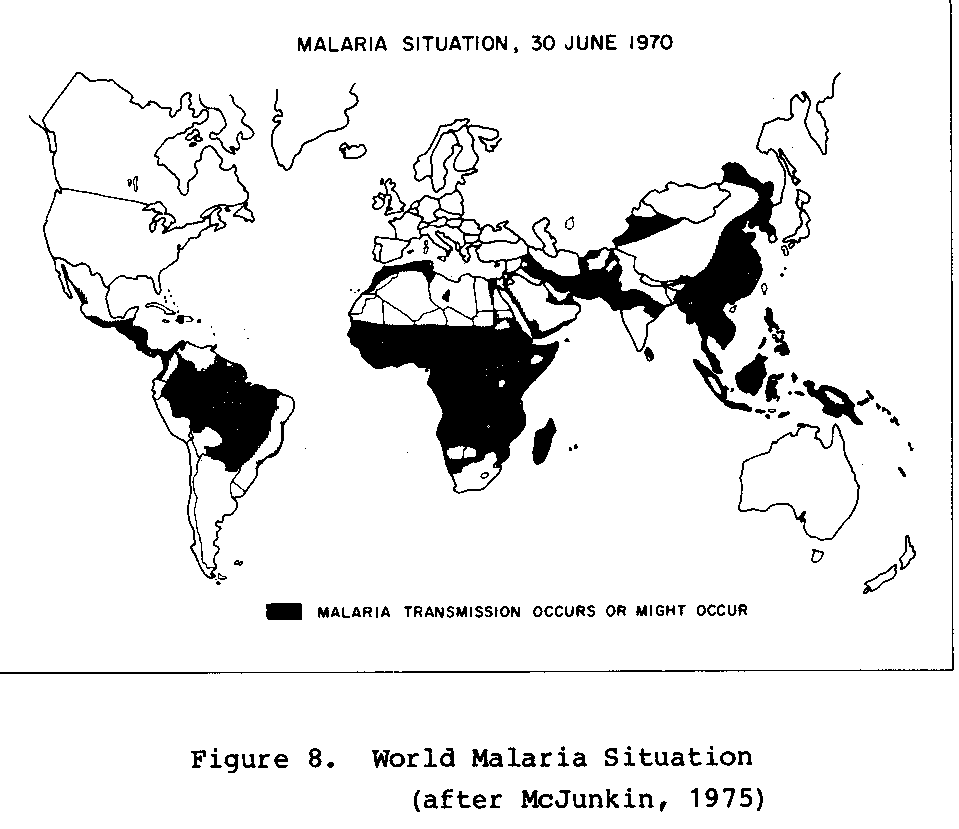
this parasite (Plasmodium falciparum, P. vivax, P. ovale
and P. malariae) cause different types of malaria,
differing in severity and timing of the malarial fevers
they produce.
The parasite is introduced into a human bloodstream
through the bite of an infected mosquito, commonly one of
the many species of Anopheles. The parasite multiplies in
the liver and the blood stream of the human host, eventually
invading the red blood cells. After being taken
into a mosquito during a blood-meal, some of the parasites
reproduce and become infective. Lodged in the mosquito's
salivary glands, they can be injected into a human on the
next blood-meal.
The symptoms of the disease are recurrent fevers and
chills plus abdominal enlargement. The disease does respond
well to several modern and classic medicines such as
quinine and atabrine. Other medicines have been discovered
that can prevent the development of the parasite,
but weekly treatment is required. However, recently,
there have been reports of more virulent forms of malaria
that do not respond to normal treatment or to prophylactic
measures.
Enteric Disease
More for the sake of simplicity than precision in medical
terminology, several actual diseases are lumped together
here under the general term "enteric disease."
Caused by
a variety of pathogenic microorganisms (viruses, bacteria,
protozoans), the diseases share common characteristics in
that they all involve intestinal disorders resulting from
ingestion of water contaminated by human excreta. The
severity of the disease depends on the type of infection,
ranging from the high mortality rates of cholera and
typhoid to the less serious but debilitating diarrheal
diseases. Bacillary and amoebic dysentery, gastroenteritis,
enteroviral and rotaviral are also included.
Symptoms vary with the specific diseases, but all involve
degrees of diarrhea, abdominal pain and fever. As we
would expect for such a broad category, the duration of
the symptoms is variable, as is the method of treatment.
What is uniform and important to the community worker is
the high rate of infection. Some experts estimate that as
much as 90% of all people living in tropical rural areas
suffer from some type of enteric disease.
DISEASE PREVENTION IN WATER PROJECTS
Since most water-related diseases do not respond well even
to expensive modern medicines and since treatment in any
case can produce side effects, the best way to control the
diseases is to prevent their spread through water
improvement
projects. Table 3 shows the potential for disease
control through various types of common improvement
projects.
To control disease one needs to know a good deal
about it and how it is transmitted. For instance, if the
disease is caused by a parasite transmitted by a non-human
vector, it is helpful to know the complete life cycle of
the parasite, the biology of the vector, and the most
likely points of contact between humans and the vector.
Within this complex arrangement, weak points in the
transmission
cycle can be identified and the environment
altered to make disease transmission less likely.
TABLE 3
PERCENTAGE REDUCTION OF DISEASE BY
WATER IMPROVEMENT
Estimated reduction by water
Disease improvement
(%)
Cholera
90
Typhoid
80
Hepatitis
(Infectious) 10?
Bacillary
Dysentery 50
Amoebic
Dysentery 50
Gastroenteritis
50
Scabies
80
Yaws
70
Leprosy
50
Diarrheal
Diseases 50
Ascariasis
40
Schistosomiasis
60
Guinea
worm 100
Sleeping
sickness 80
Onchocerciasis
20?
Yellow
Fever 20?
(after Bradley
in Feachem, 1977)
Schistosomiasis Prevention
*
Dual water systems are an excellent,
although
expensive
means to limit snail-human contacts.
Except in the
case of fishermen and irrigators, the
most frequent
human contact with water in rural
villages
involves fetching domestic supplies for
drinking and
cooking, bathing, washing clothes and
recreational
bathing, all of which may risk exposure
to
schistosomiasis. If one water supply is
developed
solely for drinking, cooking and washing
while the
other system is kept for agriculture or
waste
disposal, infection is less likely to occur.
This may be
quite simple in the moist tropics where
new water
supplies can be easily found. In fact,
in moist
tropics, catchments and cisterns can be
developed so
that potable water can be collected
with each
rainfall and protected until used. In
more arid
regions, new tubewell techniques and
inexpensive
polyvinyl chloride (PVC) pipes are
providing
uncontaminated water for village use.
*
Eliminate snail habitat. Although the
different
snail vectors
prefer slightly different habitats,
they all like
stagnant or sluggish water. Therefore,
removing
sediment and waterweeds and increasing
the rate of
flow in waterways will help control
snail
populations.
Unlined canals
or channels are more likely to
harbor snails
than those lined with concrete, plastic
or any other
material. The lining prevents
burrowing,
eliminates plant growth and improves
flow
velocities. Pipes or covered conduits are the
best but most expensive means of
eliminating snail
habitats.
*
Efficient drainage. Snails can breed in
small
areas of
standing water, such as seeps from unlined
canals,
clogged ditches or borrow pits (places
where earth
has been excavated for use elsewhere) .
Improving the
drainage of these areas will reduce
the habitat
available to the snail.
*
Physical barriers between likely snail
environments
and human
activity reduce the chance for infection.
If fences or
distance separates necessary
but
slow-moving ditches or drains from village
compounds,
humans--especially children--are not as
likely to use
the water for recreation or bathing
or to defecate
or urinate into the water channels.
*
Improve sanitation. If the eggs of the
schistosomes
do not reach
water, the cycle can be interrupted.
Improved
sanitation can reduce the number
of eggs in
snail-infested water. Simple latrines
and waste
treatment eliminate a large percentage of
the helminth
ovi reaching water bodies--though not
all.
*
Several molluscicides have been developed
for snail
control and,
if properly used, can be effective.
If the chemicals
are applied to water with high
snail
populations just before the main breeding
period, they
are quite efficient. Since molluscicides
are expensive
and their effects on other
organisms are
not fully known, their use should be
restricted to
those times and sites of high snail
densities and
at concentration specified on the
container
label.
Chemical
control with natural products is another
possibility.
For instance, in Ethiopia, an astute
observer noted
that downstream from a riverbank,
much used for
laundering, there were relatively few
snails.
Investigation revealed that a local berry
used for soap,
and appropriately named soapberry
(Phytolaca
deocodandra), contained a chemical
lethal to
snails. This area of research has been
sadly
neglected, but it holds some promise if only
development
workers and scientists could spare the
time to pursue
field studies.
*
Biological control is a preferred
alternative. In
some places it
is possible to introduce snails that
eat the
schistosome snails, but do not carry schistosomiasis.
However, this
type of program involves
trained people
who are familiar with the biology of
the different
snail species. Also, there has been
encouraging
research showing that some species of
marsh flies
have larvae that eat snails. The marsh
fly does not
bite humans and, so far as is known,
does not carry
any human diseases. Other research
has found
certain species of fish which eat snails
and could be
introduced into snail habitats. Development
of a
biological control program would require
support from a
technical assistance organization
or a local
university.
Control of schistosomiasis depends on the use of all the
available techniques. Successful control based on a
single method is very unlikely or, at best, temporary.
Nor is it likely that a complete, comprehensive control
program can be instituted at once. The development worker
must select options that are possible and implement them
as best he can--more than likely, one step at a time.
Above all, care should be taken to see that a new project
does not increase the disease through poor planning.
Onchocerciasis Prevention
Control of onchocerciasis or River Blindness is a
frustrating
problem. The disease does not respond well to medical
or chemical treatment, nor is it easily controlled by
environmental changes. The black fly vector breeds in
swift, turbulent water, such as waterfalls or rapids.
Moreover, the insect is a strong flyer, capable of traveling
more than 50 km from its breeding site. The disease
is not affected by improved sanitation or health education
programs. The only control programs involve resettlement
or application of chemicals. Where the disease is
prevalent:
*
Avoid project designs that will create
breeding
sites for
black flies, such as fast, open spillways
or turbulent
sluiceways.
*
Remove fast, turbulent waters where
possible,
through the
construction of small impoundments or
barriers.
*
Use insecticides on critical areas during
periods
of black fly
breeding or seasonal human use.
*
Insofar as possible, provide protection from
black
fly bites.
Mosquito netting or settlement at
breezy sites
may reduce incidence of the disease.
Malaria and Filariasis Prevention
Measures for effectively controlling malaria and
filariasis in water development projects depend on
elimination of mosquito breeding sites. Mosquito larvae
require stagnant water in which to mature. Extremely
small areas will suffice as breeding habitats--a discarded
food can or an abandoned tire.
*
Uncovered standing water should be
eliminated
around living
and work areas. This is especially
true in the
moist tropics where frequent rains can
produce small
but persistent pools that provide
ideal
conditions for mosquito breeding. Artificial
containers,
such as abandoned pails, barrels or
pots should
not be left to accumulate water.
*
Ditches and drains should be maintained to
permit
constant water
flow. Waterweeds provide excellent
mosquito habitat. Community efforts are
required
to remove
waterweeds from small water bodies,
ditches and
drains.
*
In arid regions, all water storage devices
should
be kept
covered to restrict egg-laying by adult
mosquitoes. An
inventory of potential mosquito
breeding sites
will almost always reveal exposed
standing water
which could be easily covered,
drained or
deepened to deny additional breeding
grounds.
*
Chemical control measures work best when the
chemicals
can be
directed at the larvae. Larvicides are
less toxic to
other animals and, when mixed with
oil, can be
applied as a thin film over breeding
surfaces.
Pesticides for adult mosquito control
are relatively toxic, more persistent and
more
costly. These
chemicals should only be applied to
homes and
public buildings at rates and concentrations
listed on
container labels. These insecticides
are too costly
and polluting to use outdoors.
Coordinated
programs of larvicide sprays on
breeding sites
and periodic spraying in dwellings
have been
effective in controlling malaria in many
communities.
Enteric Disease Prevention
The key to control of enteric disease is the disruption of
the fecal-oral route of contamination. This requires
improvements in domestic water supply and human excreta
disposal.
*
Implementing dual water systems, one for
safe
domestic water
and the other for non-potable uses,
is a preferred
method. Ground water from deep
wells or
boreholes is usually safer to drink than
surface water.
If surface water or shallow dug
wells are used
for domestic water, disinfection or
filtration
systems should be instituted (as described
below).
*
Excreta disposal methods should be developed
to
reduce or
eliminate fecal contamination of domestic
water. This
does not necessarily mean the use of
an expensive
sewerage system--merely the control of
human waste
disposal at sites where potable water
must be
protected.
*
Education programs are essential in the
control of
enteric
diseases. If people can be convinced that
these diseases
have causes that can be corrected
and need not
be a natural condition of life, they
will avail
themselves of water supplies and sanitary
measures to
reduce enteric infections. Without
an education
program and community support,
projects are
not likely to be effective.
Whether the project involves domestic or agricultural
water supplies or the development of community sanitary
stations, the project should be planned so as to avoid
increasing the level of disease in the community, and
preferably to reduce it. Wherever possible, the project
should have as an objective the reduction of a prevalent
water-related disease by a certain percentage or within a
certain age group. Given the constraints of time and
budget, this may not be realistic where irrigation is the
primary objective. Often the development worker can only
hope to maintain existing disease levels while anticipating
longer-term community health benefits from better
nutrition, higher standards of living or reduced workloads.
WATER PURIFICATION
Water for drinking and cooking can be improved at relatively
little cost and with great benefit to health. An
accurate but inexpensive chlorinator is the most effective
method for killing water-borne pathogens, but may not be
available or cost-realistic in remote, sparsely populated
areas. In these situations, no other single process can
equal the improvements in the physical, chemical and
biological
quality of surface water produced by a slow sand
or biological filter. These simple and inexpensive systems
do not require chemicals, energy or excessive maintenance.
Ideally, surface water supplies should receive
both filtration and accurately measured chlorination while
sub-surface waters require only chlorination. The slow
sand filter is encouraged since it can be constructed from
locally available material by local labor and the quality
of the water supply is significantly improved.
The essential parts of a slow sand filter are: 1) a
water-tight container (a 55 gallon barrel--200 liters--is
a good size); 2) a small amount of gravel; and 3) washed
sand (Figure 9).
esw9x43.gif (540x540)
After making sure that the container or drum did not carry
highly toxic chemicals, it should be thoroughly scrubbed
and disinfected with bleaching powder. A 5 cm layer of
clean gravel should be placed on the bottom of the drum,
covering the perforated outlet pipe. A 70-75 cm layer of
clean sand is placed over the gravel, leaving 10-15 cm to
the top of the drum. The raw water inlet pipe enters the
filter near the top. A flat rock or small dish placed
under the inlet will prevent disturbance to the sand
layer. Simple valves for the inlet and outlet pipes plus
a top cover complete the filter and it is ready to deliver
filtered water at rates of up to 1 liter per minute. The
filter will not be completely effective until the biological
layer becomes fully active, which takes a few days.
The biological layer is a thin film of algae, bacteria and
other microorganisms that develops on top of the sand and
is very important in the purification process. The
microorganisms
break down organic matter in the water and
strain out much of the inorganic particles. As the water
moves through the sand, other microorganisms continue to
remove impurities. By the time water reaches the gravel
and the perforated outlet, over 99% of the bacteria and
schistosome larvae will have been removed if the filter
has been properly maintained.
Fortunately, maintenance is a simple task. When water
flow at the outlet is noticeably reduced, it is time to
clean the biological layer. After allowing the water to
drop below the level of the sand, the biological layer,
plus a few millimeters of sand, are removed. That is the
extent of the frequent maintenance (every 2-4 weeks).
When more than half of the sand has been removed, it is
necessary to replace the sand and gravel with freshly
washed materials. This may be necessary once or twice a
year.
The slow sand filter can be further improved by two other
inexpensive additions: a settling container and an
aerator. The settling container can be another drum,
placed so that water must enter it before passing to the
filter. The suspended solids in the water held in the
first drum settle out before reaching the filter. The
absence of suspended materials prolongs the useful life of
the biological layer in the filter, thereby cutting down
on maintenance.
During filtration, oxygen is removed from the water by the
microoganisms in the filter. Water tastes better when it
has oxygen, so an aerator can be added to the filtration
system. Water coming out the drain can be aerated by
passing it over an inclined plane or series of cascades
(steps) into a storage container. Or the water can be
passed over built-in weirs as in Figure 10.
esw10x45.gif (600x600)
Another method of purifying domestic water supplies is
disinfection. The two most common methods are boiling and
chlorination. Boiling for 20 minutes does destroy most
pathogens but alters the taste of water and uses large
amounts of fuel, which is often in very short supply.
Chlorination is effective and relatively inexpensive.
Chlorine solutions can be prepared from bleaching powder
or high-test hypochlorite. To maintain the chemicals'
effectiveness, both forms should be stored in a cool, dark
place in non-corrosive containers. Stock solutions can be
made by adding 40 grams of bleaching powder or 15 grams of
high-test hypochlorite or 150 ml of liquid bleach to one
liter of water. These stock solutions can then be used to
disinfect drinking water by adding three drops of any one
of the solutions for each liter of water to be disinfected.
If the organic material in the water is high
enough to color the water, the dosage should be doubled.
The water should be mixed and allowed to stand for 30
minutes before use.
Simple chlorinators can be devised from local materials to
purify well water.
a. Single pot chlorinator (Figure 11). A 12-15 liter
esw11x46.gif (600x600)
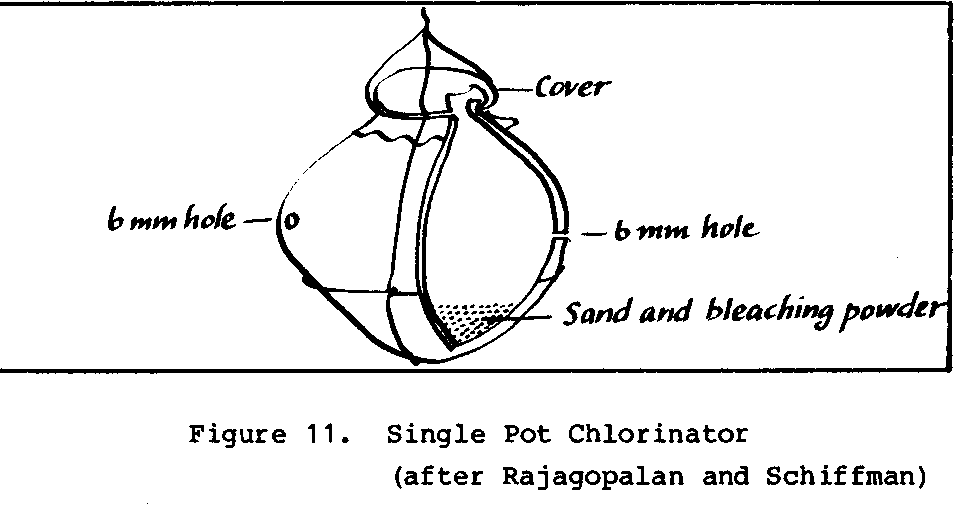
earthen pot with
two 6 mm holes is filled with a
mixture of 1.5 kg
bleaching powder and 3 kg coarse
sand. After the
top has been fitted with a
water-tight cover
(rubber or polyethelene), it is
suspended 1 meter
below the low water level of the
well. The
chlorinator will disinfect a well which
yields up to 1200
liters per day for seven days.
Another version of this can be made with a large
coconut, split horizontally and hollowed out.
Three .5 cm holes are burned or bored midway down
the lower portion of the shell. A plastic bag
with 1 kg each of sand and bleaching powder is
sealed and two .8 cm holes are made near the top.
The bag is placed in the coconut and the halves are
closed with twine. The shell is hung 30 cm below
water level and can disinfect yields up to 90
liters per day for about 3 weeks.
b. Double pot
chlorinator (Figure 12). This type,
esw12x47.gif (600x600)

which is more
effective for a longer period, consists
of a container
filled with a 1 kg of bleaching
powder and 2 kg
of sand placed inside a second
container. The
inner container should have a 1 cm
diameter hole
approximately 3 cm above the sand-bleaching
powder mixture.
The larger container
should have a
water-tight cover and a 1 cm diameter
hole about 4 cm
above the bottom. The chlorinator
is placed 1
meter beneath the water surface. Most
family wells
(under 5000 liters) can be disinfected
for 2-3 weeks
with this chlorinator.
Table 4 provides guidelines for the amounts of chlorine in
alternate substances which could be used to disinfect
large amounts of water for domestic use. Remember, these
are guidelines for water with "average" amounts of
organic
material and tests would have to be done to determine if
the amount is correct for a specific water supply.
TABLE 4.
AMOUNTS
OF CHLORINE NEEDED TO DISINFECT DRINKING WATER
Water
Bleaching
High Strength Liquid
Bleach
PLUS
Powder
OR Calcium
OR
(5% Sodium
Hypochlorite
Hypochloride)
1 cubic
meters
2.3 grams
1 grams
14 milliliters
1.2
PLUS
3 OR
1.2
OR 17
1.5
3.5
1.5
21
2
5
2
28
2.5
6
2.5 35
3
7
3
42
4
9
4
56
5
12
5
70
6
14
6 84
7
16
7
98
8
19
8
110
10
23
10
140
12
28
12
170
15
35
15
210
20
50
20
280
30
70
30
420
40
90
40
560
50
120
50
700
60
140
60
840
70
160
70
980
80
190
80
1100
100
230
100
1400
120
280
120
1700
150
350
150
2100
200
470
200
2800
250
580
250
3500
300
700
300
4200
400
940
400
5600
500
1170
500
7000
(after Ram 1979)
Neither the simple filter nor the primitive chlorinators
will make the drinking water absolutely safe for drinking,
since even the most modern systems cannot totally eliminate
the transmission of water-borne disease. However,
these simple devices will make the water safer at low cost
and, to the world's rural poor, these are the most important
considerations.
For projects in larger villages with more resources,
planners
may select low-cost chlorinators which are available
and are much safer. These new chlorinators are reliable,
durable and require minimum attention for operation and
maintenance.
The decision as to which water treatment system is most
appropriate will depend on the number of people to be
served by each unit and the amount of funds available.
4.
ENHANCEMENT, DEVELOPMENT AND PROTECTION <see image>
esw4x50.gif (486x486)
"Water is the driver of
life."
Leonardo da Vinci
The goal of water projects is most often to increase the
amount of water available to a community. The supply can
be increased in several ways:
1.
Make more efficient use of existing water
supplies. In
most existing systems, a large amount
of water is
wasted through unwise use or poor
methods of
collection.
2.
Improve water delivery systems to reduce
water
losses
through evaporation or seepage.
3.
Enlarge existing sources.
4.
Develop new sources of water.
5.
Protect watersheds and other sources of
water in
order to
maximize water output and avoid wide
fluctuations.
The list given here is arranged in order of priority for
the community worker but not strictly by environmental
preference. The fifth alternative is the most ecologically
sound approach to water management. Water projects
have a better chance of gaining community support if the
benefits are immediate and apparent. Watershed protection
or improvement projects are long term; benefits are
indistinct or accrue to future generations. Therefore,
these projects are less likely to receive enthusiastic
support unless an education program can persuade the
community of their present importance and future value.
The first three alternatives receive high priority because:
1) community benefits are immediate and apparent;
2) investment is relatively low; 3) environmental impacts
are not significant; and 4) cultural adjustments are
minimized.
When there are deficiencies in community water supplies,
marked either by insufficiency or high rates of waterborne
disease, the first reaction is generally to locate
and exploit a new source of water without first considering
improvement of the existing system. For economic,
social and environmental reasons, community workers should
reject proposals for new sources until the first three
alternatives (above), either singly or in combination, are
judged to be insufficient or unsound. Indeed, all
alternatives
should be examined before selecting the best method
for increasing the water supply to meet the desired
objectives.
CALCULATING AND USING WATER CAPACITY AND CONSUMPTION
The first step in the process is to calculate the water
requirements of the community and measure the capacity of
the existing system.
In small villages with a limited number of water sources,
such as community wells or standpipes, actual water
consumption can be measured by recording the amount of
water taken from each source over a period of two or three
days. From these data, annual water use can be estimated.
It is important to remember that if water is made
more readily available, annual consumption is likely to
increase. Another method is to employ minimum standards
for domestic water use proposed by various international
organizations. For instance, UNICEF recommends a daily
per capita minimum of 38 liters for drinking, cooking and
bathing. This figure multiplied by the community population
would provide an estimate for domestic water requirements.
For agricultural or commercial water supplies,
estimates can be gained from the technical literature or
from technical advisors, if available.
Evaluating the capacity of the existing system requires a
little field work and investigation. Each community water
source should be mapped and notes taken on capacity and
quality, as well as the type of use for each source, e.g.,
drinking, washing or agriculture. The yield of the spring
can be determined by measuring the time required to fill a
container of a given volume. Yields from wells can be
measured by bailing or pumping a given volume after marking
the initial water level. The time required for the
well to return to the original level is then used to
calculate the yield per unit of time. The volume of water
in a stream or channel can be estimated by measuring the
cross-section area of the water flow and calculating the
water velocity by timing a float along a measured distance.
VITA's Village Technology Handbook and Peter
Stern's excellent reference, Small-Scale Irrigation, (see
Appendix I) provide assessment methods for each water
source.
Planners should not overlook the educational opportunities
in the mapping and measuring exercises. Local teachers
could use the occasion for teaching math, public health or
mapping. Student involvement will also increase the level
of community participation, since the students can be
expected to relate their experiences and lessons to their
parents.
Of course, account must be taken of seasonal variation in
the water sources. Village elders can provide the necessary
information for this adjustment to the estimate. The
elders will know which wells run dry and for how long and
can describe the low water flows of streams and channels
during the dry seasons. Unless storage facilities are
available, the dry season capacity should be used in
evaluating
the project alternatives.
If community water supplies are greater than water
requirements, as is often the case in tropical moist
areas, then the real question may be how to protect the
water from pollution or how to use it wisely. If the wet
season capacity exceeds community requirements and there
is a shortage in the dry season, then the best water
development
project may be some type of storage system so as
to use the yearly water supply more efficiently.
In arid regions, the maximum seasonal water supply may be
adequate or barely adequate; for the rest of the year
outputs may fall well below minimum requirements. If
minimum consumption requires more than a 100% increase in
water supplies, it is likely that new sources will be
needed. Otherwise, the objective may be achieved through
a water conservation program, improving delivery or storage
systems, or enlarging existing sources. In fact,
these three alternatives are productive even if the
development
of new sources is ultimately necessary.
COMMUNITY WATER CONSERVATION PROGRAM
The most obvious waste of water is from a source that runs
constantly whether in use or not. Water is also wasted
when the flow cannot be constrained to conform to the rate
at which containers or conduits can be filled. Water
sources should be equipped with devices, such as valves or
reduction pipes, that can vary the flow and force of water
being delivered.
Excessive use of water is most common in agriculture,
where farmers tend to put out more water than livestock
require or to irrigate with larger quantities than are
needed by the plants for optimal production.
Evaporation and seepage losses can be reduced to provide
more available water. Exposed water surfaces, whether in
reservoirs or ditches, lose huge quantities of water.
Covers for water storage units or ditches can reduce
evaporation
up to 50%. By digging reservoirs and ditches
deeper, more water can be moved or stored with less surface
area exposed to evaporation. Seepage can also account
for immense losses. Dug wells, springs or unlined
irrigation canals allow water to seep into the soil.
Lining with impervious materials like concrete or rocks
can reduce the loss substantially. If pipes rather than
open unlined canals are used, the water that can be
delivered
is effectively doubled simply by reducing losses.
In addition, lined canals or closed pipes do not provide
good habitat for snails and mosquitoes.
Conversion to closed water systems can be programmed over
several years as labor, materials and funds are available.
Each section of open canal or storage system that
can be covered or lined will reduce water losses, until
the system has been completely converted.
IMPROVED DELIVERY OR STORAGE SYSTEMS
Often, the water supply for a community would be adequate
if it were evenly distributed over the year. Where it is
not, improved storage systems can capture more water when
it is available, for times when it is scarce.
The construction of enclosed water storage containers or
cisterns can be quite effective. The Village Technology
Handbook provides construction details for several types.
Cisterns require a collection area or catchment to gather
water during the wet season. A common method, used in the
Caribbean, is to collect rain from the roof, allowing it
to drain into a covered tank (Figure 13). Later the water
esw13x56.gif (353x353)
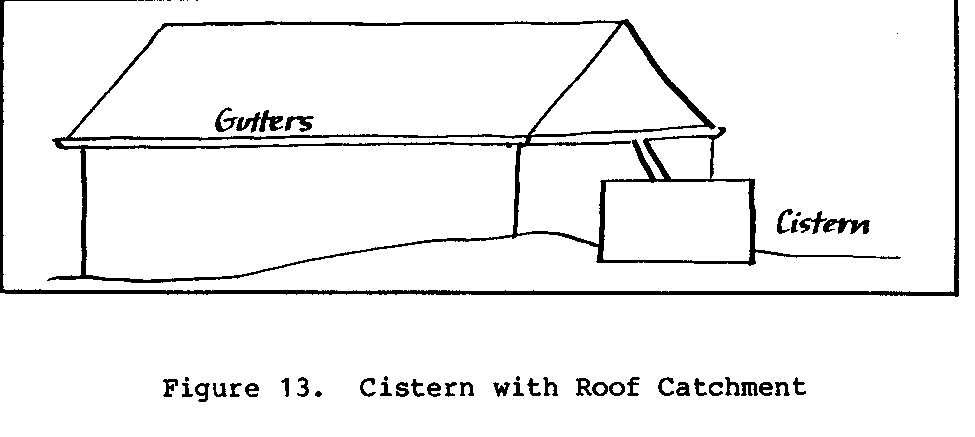
can be purified with small amounts of chlorine.
Catchments for agricultural cisterns were a common practice
in the arid Middle East. Channels or furrows were
placed along barren hills leading to large cisterns or
open reservoirs at the base of the hill. Surface water
running off the hill was diverted by the water channels
into the reservoir or cistern (Figure 14). This practice,
esw14x57.gif (393x393)
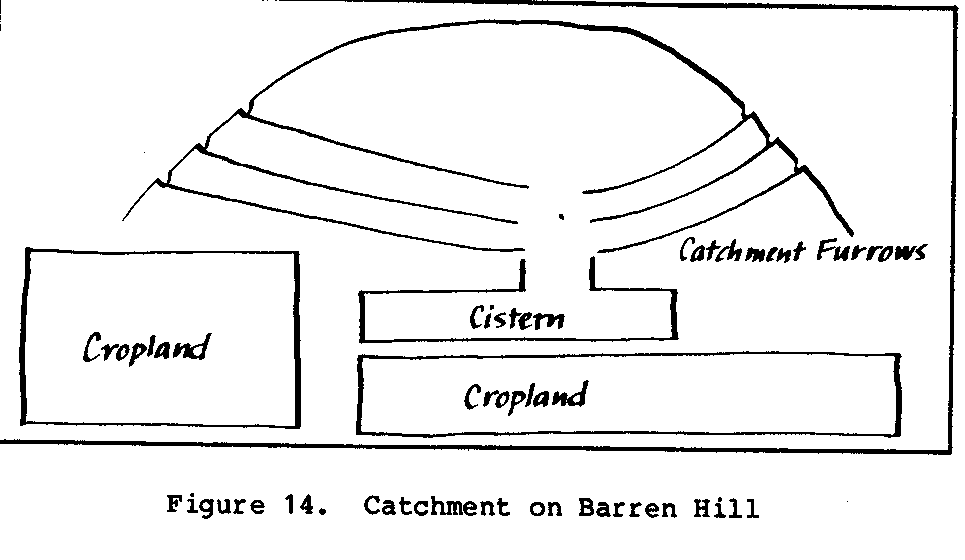
over 2,000 years old, is being reintroduced in Israel with
excellent results. During the dry season, the catchment
can be improved by compacting the furrows or lining the
catchment channels with rocks, concrete or plastic
membranes.
Asphaltic concrete has been applied to entire
catchment areas, so as to capture even more of the precious
water. The National Academy of Sciences devotes an
entire chapter in its book More Water for Arid Lands to
domestic and agricultural catchments. (See Appendix I.)
In all types of storage systems, closed cisterns are
preferred
to open reservoirs. By enclosing the storage tank,
evaporation and seepage losses are kept at a minimum and
the tanks do not provide breeding habitat for snails or
mosquitoes. The cisterns should be placed as close as
possible to the point of use to minimize the expense and
complexity of delivery systems. In community systems, the
roof area of several houses can feed into one cistern,
thereby reducing the costs of construction.
Often, safe water is available to a community only at
considerable distances, requiring the women and children
to walk many kilometers several times per day to secure
household supplies. If the burden becomes too great, they
may turn to a source that is unsafe, but nearer by.
Improving the delivery system is always an expensive task
unless local labor and materials can be used. The advantages
of lining or piping are apparent, but heretofore the
high cost of concrete, fiberglass or polyvinyl chloride
(PVC) has been prohibitive. Recent improvement and transfer
of PVC technology provides exciting new possibilities.
In countries where the technology is available, the
light and flexible PVC pipe is relatively inexpensive and
adaptable to' rural conditions. Many community systems
have been built with PVC pipe using local labor to dig
trenches and to transport the durable piping.
Obviously, there are prerequisites to piped water systems.
Unless the water source is at a higher elevation
than the village, expensive pumps are necessary. The pipe
must be buried, since sunlight makes the material very
brittle. Unfortunately, rodents seem to be attracted to
PVC, often gnawing small holes in the pipe. Frequent
inspection and repair of the system is therefore necessary.
The use of PVC is expected to increase as more
developing countries acquire the pipe-making facilities.
Concrete is more commonly used for linings or conduits in
developing countries. The advantages of concrete are that
it can be cast on-site and local labor can easily develop
the necessary construction skills. The Village Technology
Handbook provides technical information plus designs for
forms and molds that can be used for casting linings or
conduits for water supply systems.
Concrete lining permits construction of narrow and deep
canals with higher water velocity to reduce mosquito and
snail breeding and less surface area to minimize
evaporation.
Water flow is more easily regulated in pipes or
lined canals so less water is wasted through uncontrolled
flows. Seepage losses in both situations are virtually
eliminated.
In tropical moist areas, wood conduits may be used to
carry water. Bamboo makes excellent piping, where small
water volumes are handled. The villagers will know how to
remove the internal barriers in the bamboo. The ends can
be shaved or widened to form water-tight connections, once
wrapped with tarred rope. The bamboo is light, sturdy and
somewhat flexible--a good piping material for bringing
water from spring or cistern to a dwelling or central
square.
ENLARGING EXISTING SOURCES
Any water source unattended for a number of years has been
reduced in efficiency and capacity by the addition of
debris and sediments. Dug wells and tube wells slowly
fill in as walls erode. As these wells are cleaned, they
can also be enlarged either in depth or diameter. With
lined wells, or tube wells with casings, increasing the
diameter is not recommended, since the lining would have
to be removed. However, the well can be enlarged below
the lining or casing and, unless there is impervious rock
layer, can be dug deeper.
Open reservoirs and surface-water cisterns accumulate
sediments and require periodic cleaning. The reservoirs
can be enlarged during cleaning by removing more material
both in and around the reservoir. Care should be taken
not to disrupt any impervious layer or to exceed the water
volume that can safely be held back by the dam or barrier.
DEVELOPING NEW WATER SOURCES
If all the alternatives for improving existing sources
have been explored and a community is still short of
water, the search for new sources should be undertaken
with recognition that the easy or obvious may have already
been done; new sources may be harder to find or more
expensive to develop. Some possibilities--such as
cisterns--have
been discussed under the heading of improvements.
The most likely new source will be various kinds
of wells.
*
In rural areas, dug wells are a very common
method
of providing
new water. If lining materials such
as concrete or
masonry are available, dug wells are
inexpensive
and durable. Wells 1.5 meters in diameter
can be dug by
two men, and sections of the
concrete
casing can be cast in place. After the
first section
is cast, the well-diggers merely
remove soil
beneath the casing and, as it is
lowered, new
sections are cast on top until the
well goes
below the dry season water table.
Linings are
recommended for dug wells to prolong
well life and
to reduce contamination. The casing
or lining
should rise at least one meter above
ground level.
This prevents small children and
animals from
falling into the well and also reduces
the amount of
contaminated water spilling back from
the ground
surface.
Ideally, the
well should be covered and water
removed by
some type of hand pump, rather than
expensive and
hard-to-repair motorized pumps.
Realistically,
however, in many remote areas even
hand pumps
create insurmountable repair problems
and the most
feasible well design incorporates
simple bucket
mechanisms. The bucket should be a
part of the
well so that people do not use their
own
containers, which may be contaminated, to dip
water. Several
bucket designs, including self-tipping
varieties, are
available in appropriate
technology
literature.
*
In areas of soft soils, sand or limestone,
bored
wells can be
made with hand-powered borers or
augers. If and
when water-bearing layers are penetrated,
small diameter
pipes with strainers are
sunk into the
bore hole and a pump is attached.
The well should
be capped to prevent contamination.
The Village
Technology Handbook offers
directions on
how to construct simple boring equipment
and advice on
techniques. The book also has
technical
designs for concrete tile machines to
make well
casings. Bore or tube wells are commonly
used for
domestic and agricultural water supplies.
If soils are
favorable and the boring equipment is
available, the
bored wells are an environmentally
sound source
of water, providing non-motorized and
easily
maintained pumps can be used.
*
Driven wells are made by driving a
well-point (a
pointed
strainer) into soft or sandy soils. The
well is driven
by pounding pipes (with a special
cap) connected
to the perforated well-point until
water-bearing
layers are reached. The pipes provide
a well casing
as they are driven into the
ground. The
driven wells require a special pump
and this may
produce maintenance problems.
A problem
common to all types of wells is seepage
of
contaminated water back into the source. Even
the best
casings cannot prevent all seepage, so
methods to
reduce spillage or seepage must be
devised. Wells
should be surrounded by sloping
concrete
aprons with drainage furrows. Water
spilled at the
well will then be directed away
rather than
back toward the casing. Trenches of
loose gravel
around the edge of the apron will
eliminate
puddles which encourage snail or insect
breeding.
Layers of clay mixed with water puddled
around the
casing will also reduce seepage into the
well (Figures
15 and 16).
esw15x62.gif (594x594)
To keep
livestock away from wells, water should be
carried by conduits to troughs or holding
tanks
some distance
away. Gravel beneath and around the
trough and
tanks eliminates standing water.
People can
also be encouraged to do laundry or
bathe away
from the well by the addition of a
cement laundry
or bathing pads 20-25 meters from
the well.
Gravel drains should also be included in
these areas.
*
Sand reservoirs can be constructed in arid
regions
to provide new
sources of water. A low barrier
(approximately
1 meter) can be built across seasonal
streams. If
the sediment load of the stream is
sand, it will
settle behind the barrier. The dam
can be raised
in one meter increments for the next
3-4 years, if
topography permits. Water will remain
in the spaces
between the sand grains and can
be tapped with
driven wells (Figure 17). Sand
esw17x63.gif (540x540)
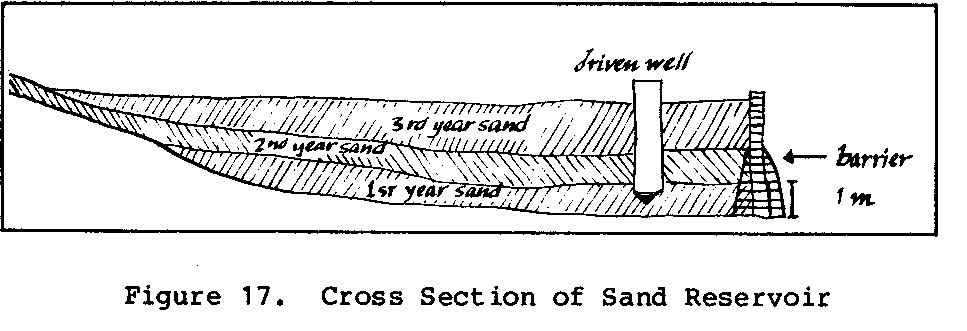
reservoirs can
hold a great deal of water without
excessive loss
from evaporation and they do not
provide habitats for disease vectors.
*
Open reservoirs are not a preferred
alternative in
arid zones
because evaporation is excessive and
health risks
are serious. However, open catchments
can serve as
temporary livestock watering areas.
Also, open
reservoirs can be useful in recharging
aquifers in
order to raise the water table and
thereby refill
dug wells.
PROTECTING WATER SUPPLIES
Once water sources are developed, they should be protected
from damage by humans and animals. Burying pipes or
building fences around wells can be helpful. The value of
these protective measures can easily be demonstrated.
However, protection of the watershed is often neglected
because the value is not readily apparent. Watershed
protection
is a long-term investment that only gradually
improves the water supply. It is difficult to see that
many current water problems are the result of poor watershed
management in the not-so-distant past.
If, in arid regions, surface water is allowed to escape as
surface runoff before it can be utilized, a major loss has
occurred. Natural ecosystems prevent excessive runoff and
erosion by providing a vegetation buffer between rain and
soil. In order to maintain this natural protection, plant
cover must be retained in water collection areas or areas
adjacent to water supplies. The vegetation can consist of
a mixture of grasses, shrubs and trees. The first step in
developing this protection is through reforestation
projects.
Young trees can be planted and tended until roots
reach deeper water layers. As the trees begin to bind the
soil and provide shade, other types of plants may be
introduced artificially or naturally, until a complete
mixture of grasses, shrubs and trees is established, providing
three plant layers of soil protection.
Water development projects should include strategies for
protection by some form of plant cover in the watershed.
The first step is education designed to convince the
community
of the necessity of protecting water sources with
plants. If the community does not understand or appreciate
the need, the project will fail.
In order to protect watersheds it is not necessary to
create inviolate preserves, but rather a management area.
Plants or wood products can be harvested as long as the
natural rate of replacement is equal to or greater than
the amount harvested. However, the management area should
be well-defined and should receive higher levels of
protection
than areas that do not contribute to the water
supply.
On watersheds unprotected by vegetation, development of
water resources must begin with afforestation, whether
with quick-growing trees or other kinds of plants. The
species of trees to be planted can be selected with the
advice of government forestry experts. However, trees
should be selected on the basis of utility to the community
as well as characteristics of quick growth for watershed
protection. For instance, fruit or nut trees can
perhaps be planted to supplement food supply or develop a
cash crop. If fuel is scarce, trees can be selected for
their value as firewood. The key to the success of tree
planting is
maintenance of their future growth. It will
not suffice to plant hundreds of trees and fail to monitor
their progress or provide them with some degree of
protection.
Even if properly planted, most trees wil not
survive unless they receive added care for a few years.
Seedling trees are vulnerable to damage by animals and are
susceptible to drought until the root systems become
established. A tree planting program must include provisions
for temporary protection from animals with barriers
or fencing and may need supplemental water for at least
two years. If the community supports the project, "tree
guardians" can be appointed to care for the trees and
to
supply small amounts of water when the symptoms of drying
become apparent.
The rewards of a well-executed watershed management program
are not dramatic. Unless records are kept, the
improvements are so subtle that villagers will not see the
results nor remember the conditions before the project
began. Like most environmental change, increments are
small and spread over long periods but they can produce
significant results. Efforts to protect watersheds are
one of the best investments for the future.
OPERATION AND MAINTENANCE
Improving or creating water supplies is gratifying, but is
only one-half of the project. Unless provisions are made
to ensure effective maintenance, the water supply may
quickly return to pre-project levels yet be expected to
fulfill an increased demand. Plans and organization for
operation and maintenance should be established before the
project begins. Community support and acceptance of
responsibility
for the project are as crucial to maintenance
as to development. Water development projects in which
villagers were assigned or elected as titled caretakers or
watchmen trained in operation and maintenance have had
great success. A community organization to "care for
the
water" can benefit water projects. Effective water
projects
will involve development of new skills and experience
in working with metals, masonry, pumps and in
preventative maintenance where appropriate. This will
contribute to sustaining the project after the departure
of the development team.
Local technology should be the basis of the project. If
the project depends on outside skills or foreign parts, it
will be only as good as the availability of those goods or
services. Before accepting a pump or other mechanical
device, make sure the replacement parts are available and
repairs can be made by members of the community.
Increasing or improving water supplies is a difficult and
expensive task. The United Nations' goal of providing
safe water for everyone by 1990 seems out of reach. But
to relieve the daily lives of those people to whom 38
liters per day would be a luxury is an achievement not to
be measured in numbers alone. Small steps in water
development
taken in thousands of villages are far more effective
than gigantic projects at a few sites.
5. SANITATION AND WASTE TREATMENT <see
image>
esw5x68.gif (353x353)
"In many instances, it is a question of
life
or death, not speak of human dignity
and
self-respect. One cannot teach a
child to read if he is debilitated by
diarrhea, or expect a man to take a great
interest in improving his shelter if he
has
to wade through his own, his neighbors'
and
his animals' filth."
Grava
In all of the nations of the world, one common problem is
adequate sanitary waste collection and excreta disposal.
However, excreta should be regarded as a resource rather
than a waste. In many parts of the world, human excreta
is regarded as a valuable commodity, carefully collected
and sold for fish farming or agriculture. However, many
health hazards are associated with these practices. In
other areas, human excreta and other organic wastes are
used to provide domestic energy supplies while satisfying
the seven performance criteria of Wagner and Lanoix.
The problems of collection and disposal are particularly
far from being solved in developing nations. Improvements
in excreta disposal are essential in order to raise levels
of public health. Small-scale waste disposal technology
has lagged behind the recent advances in water supply.
Yet, both technologies are equally important, for if water
supply is increased and sanitation is not improved, the
new water supply provides a vehicle for the further spread
of disease. Often the typical response to waste disposal
problems is to apply industrialized technology which is
expensive and wasteful of natural resources. Water-borne
sewer or septic systems, which work well in temperate
zone industrial nations, are often inappropriate in tropical
areas. Fortunately some efforts have been made
recently to determine which small-scale disposal
technologies
are cost-effective for the rural tropics.
OBJECTIVES FOR EXCRETA DISPOSAL SYSTEMS
The 1958 World Health Organization publication by Wagner
and Lanoix (Appendix I) is still the best source of
information
on the disposal of human wastes in developing countries,
although the Village Technology Handbook offers
many of the same designs and recommendations. Wagner and
Lanoix offer seven rather stringent criteria for any excreta
disposal system:
*
The system should be simple and inexpensive
in
construction
and operation
*
Handling of fresh excreta should be kept to
a
strict minimum
*
Excreta should not be accessible to flies or
animals
*
Contamination of wells and springs should be
prevented
*
Pollution of surface water should be
safeguarded
against
*
The surface soil should not be contaminated
*
There should be freedom from odors or
unsightly
conditions.
These criteria have been listed in order of priority,
although some may disagree with the arrangement.
Surprisingly,
if some of the conditions can be met, the others
fall into place or are met at least in part. In addition
to these criteria, the system must be culturally acceptable
and supported by the community. The collection,
storage and treatment of the water must not be incompatible
with local customs or religious practices.
BASIC WASTE DISPOSAL METHODS
The many methods of waste disposal are all variants of
three basic types:
*
Removal, where excreta is collected and
transported,
either
manually or automatically to a
discharge site
or a central facility for further
processing. A
common method in urban areas.
*
Infiltration, or the absorption and
dispersion of
waste
materials into soil or ground water. Common
in rural areas
and a source of serious contamination.
*
Destruction, where excreta and other wastes
are
converted into
useful and harmless substances.
The relationship of the three methods is diagramed in
Figure 18.
esw18x71.gif (486x486)
Other than bucket latrines, the most common excreta disposal
systems are combinations of the three basic methods.
Using combinations of new and old techniques may be the
best approach in designing small-scale village sanitary
systems, because one method is not likely to meet economic,
social and environmental criteria for an entire village.
A slight difference in soil texture, ground water
or proximity to surface water or cropland in one location
can make a method less suitable than it might be at
another location.
Removal systems with pipes or conduits for human wastes
are generally too costly for rural projects. In urban
areas, where large sewer mains are available for connection,
piped systems may be very appropriate. Sewer systems
are capital intensive and require large volumes of
water for operation, rendering them less suitable for arid
regions.
Removal by bucket is a common and viable option for excreta
disposal. This is especially true in villages and
cities near agricultural areas where human excreta, or
night soil, has been traditionally used to fertilize
croplands.
In many Asian communities, collectors pay householders
for the wastes which are then transported in tank
trucks or push-cart vaults to farm areas and sold to
farmers. The system is inexpensive and does not require
water; but it may create health hazards from handling or
exposure to flies and also is odorous. In the best of the
bucket systems, the bucket is routinely cleaned and tarred
or disinfected and equipped with a tight-fitting
vermin-proof
cover.
In more urbanized areas, a ventilated vault may replace
the bucket. The vault is pumped out by a vacuum truck and
the excreta taken to a central site for treatment or
directly
into the country to be sold to farmers. The system
is an improvement over buckets in that handling and odors
are reduced.
The chemical toilet is also a modification of the bucket
latrine. Bacteria-killing chemicals, such as formaldehyde,
are added to the bucket to reduce odors and handling
hazards. The initial cost of the chemical toilet is
low but depends on a constant supply of expensive chemicals.
Furthermore, the chemicals may cause environmental
harm at disposal sites by killing fish and vegetation.
The chemicals also destroy natural bacteria in the excreta
and delay the decomposition process. The chemical toilet
is not recommended as a means for excreta disposal in
rural areas.
The aqua-privy (Figure 19), a removal/infiltration
technique,
esw19x73.gif (540x540)
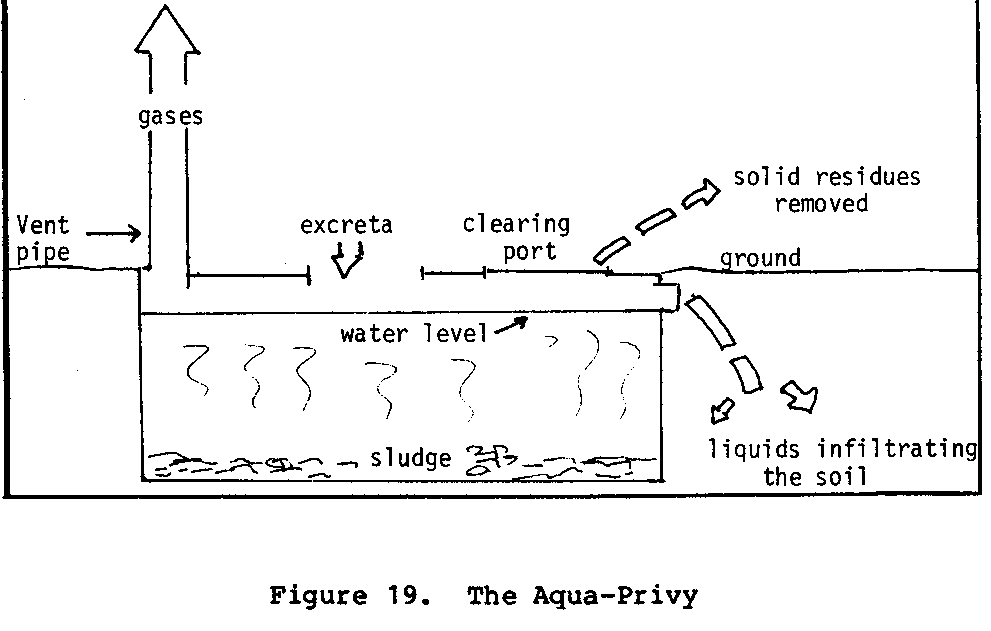
eliminates some of the problems of bucket latrines
but at increasing cost. The unit consists of a watertight
vault or tank in which water is kept at a constant
level by an overflow drain. Waste material, dropped beneath
the surface of the water, is decomposed by anaerobic
bacteria (bacteria that do not require oxygen), which are
normally present in fecal material. The resulting sludge
must periodically be removed (by pumping or vacuum
truck). If the sludge is not exposed to fresh pathogens
for three weeks, it can be spread on croplands with little
health risk. The overflow liquids, mostly urine and
contaminated
water, infiltrate the soil and may introduce
pathogens, so aqua-privies should not be located within
15-20 meters of a well or other domestic water source.
The soil surrounding the aqua-privy should be permeable to
allow rapid percolation of the effluent. To prevent too
heavy concentration of the effluent, units should be at
least 15 meters apart.
The reduction of health hazards and odor may be worth the
increased cost of the aqua-privy, if construction material
is locally available. Unless vents and other openings are
screened or covered, insects can use the water for breeding.
The vents are necessary because anaerobic decomposition
does produce a combustible gas. The vents should not
be placed near open flames and should extend at least two
meters high. In some systems, the gas can be captured for
domestic cooking and heating.
The septic tank is another removal/infiltration approach,
direct from the temperate industrialized areas (Figure 20).
esw20x75.gif (486x486)
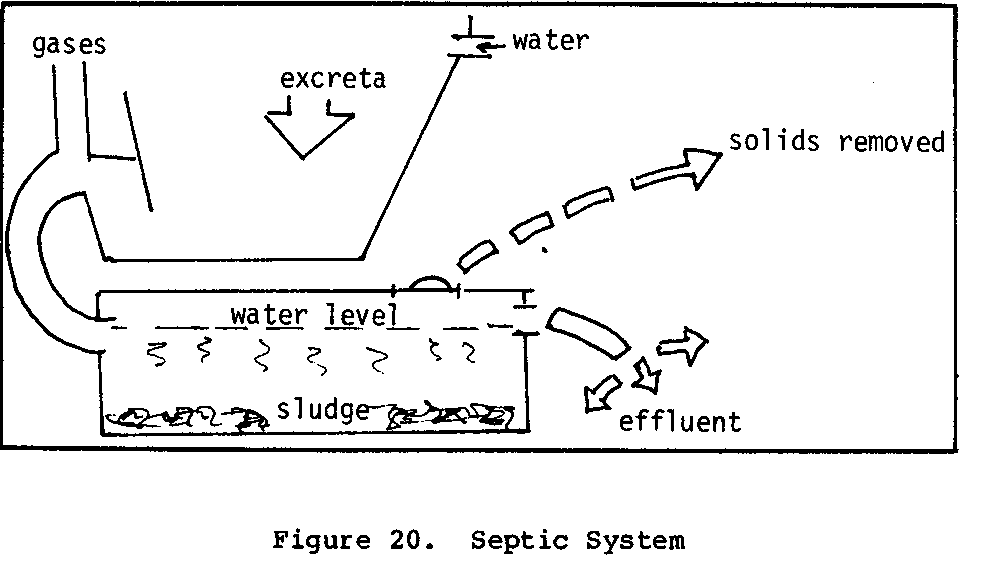
In this system, water is added to the excreta before
entering the infiltration tank. Effluent infiltrates the
soil by way of an overflow drain which can go directly
into the soil or be directed to a soakage pit or filter
drains. Solids are retained in the tank and slowly
decompose anaerobically. The residue, or sludge, must be
removed when the tank becomes filled with solid material.
It is much the same as an aqua-privy, except for much
higher water consumption, a serious disadvantage in arid
regions. The septic tank requires permeable soil or an
extensive drain field of gravel and pipes. In areas of
high water tables or impermeable soils, the effluent may
rise to the surface, providing breeding habitat for
disease vectors.
The pit latrine, which may be nothing more than a hole in
the ground, is commonly used as a temporary waste disposal
method or as the first step in the development of village
sanitary systems. With improvements, the pit latrine can
be less temporary and more sanitary.
This cheap and simple latrine consists of a hole or
trench, often covered by a plate or slab. Liquid wastes
seep off into the soil and the solids accumulate, slowly
decomposing, until the pit is filled. Thereafter, a new
pit is dug and the old one is covered with earth.
Designs for the latrine slab can be found in Village
Technology Handbook and the publication by Wagner and
Lanoix. The pit latrine does have drawbacks, to be sure.
Some soil is contaminated by the wastes and nearby water
supplies may be polluted. Latrines should not be located
near a water source. The problems of odor and flies are
not solved by pit latrines. Squatting plates can be
provided with covers, but they may be left open.
Self-closing
covers have not been successful, although several
designs are available. Squatting plates can be made of
wood, bamboo or concrete. Designs for squatting plates
with construction directions are provided in Village
Technology Handbook.
The newest innovations in small-scale waste disposal use
destruction methods. Destruction is a poor term because
the aim is to produce something useful. Incineration is
an exception since burning of wastes requires expensive
equipment without provisions for capturing the released
energy. For small-scale projects, incineration is not
recommended. Any of the following is a better alternative.
The "dry composting" techniques not only destroy
wastes
but provide an inoffensive, stable soil conditioner. The
dry composter, or Multrum, consists of a water-tight
container equipped with air intake and ventilation ducts
and two access chutes, one for excreta and the other for
organic kitchen wastes (Figure 21). Before operation, a
esw21x77.gif (486x486)
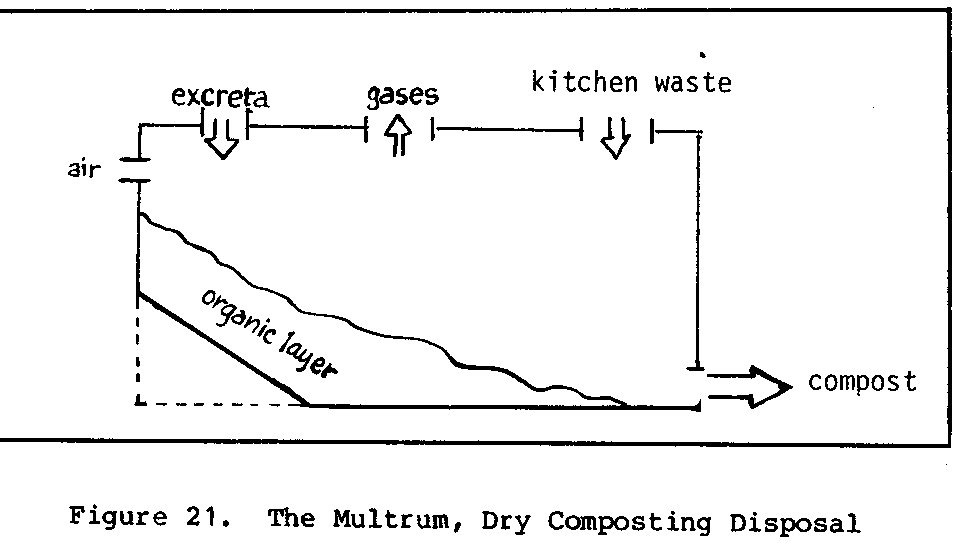
layer of partially decomposed organic material (leaves,
grass or sediments) mixed with soil is placed on the
bottom of the container. Aerobic bacteria (bacteria that
require oxygen) in the partially decayed material, along
with those in the excreta, decompose the contents into an
enriched humus which can be added to garden soils. Water
and carbon dioxide, by-products of the decomposition,
escape through the vent. As the waste settles down into
layers, it is reduced to less than 10% of the original
volume. The bottom layer, or compost, is fully decomposed
and can be removed through a small door. Heat generated
from the decomposition destroys most pathogenic organisms
including roundworm eggs and bacteria. Covers and vent
traps should be used to keep insects away from the top
layers of the tank.
The Multrum dry composter meets the performance criteria
for waste disposal systems. Initial costs are high but,
if the value of the recycled nutrients is subtracted from
the original costs, the cost/benefit ratios become very
favorable. Dry composting is simple and economical and is
one of the most ecologically sound techniques for human
waste disposal.
In Viet Nam, a double vault composting latrine (Figure 22)
esw22x78.gif (600x600)
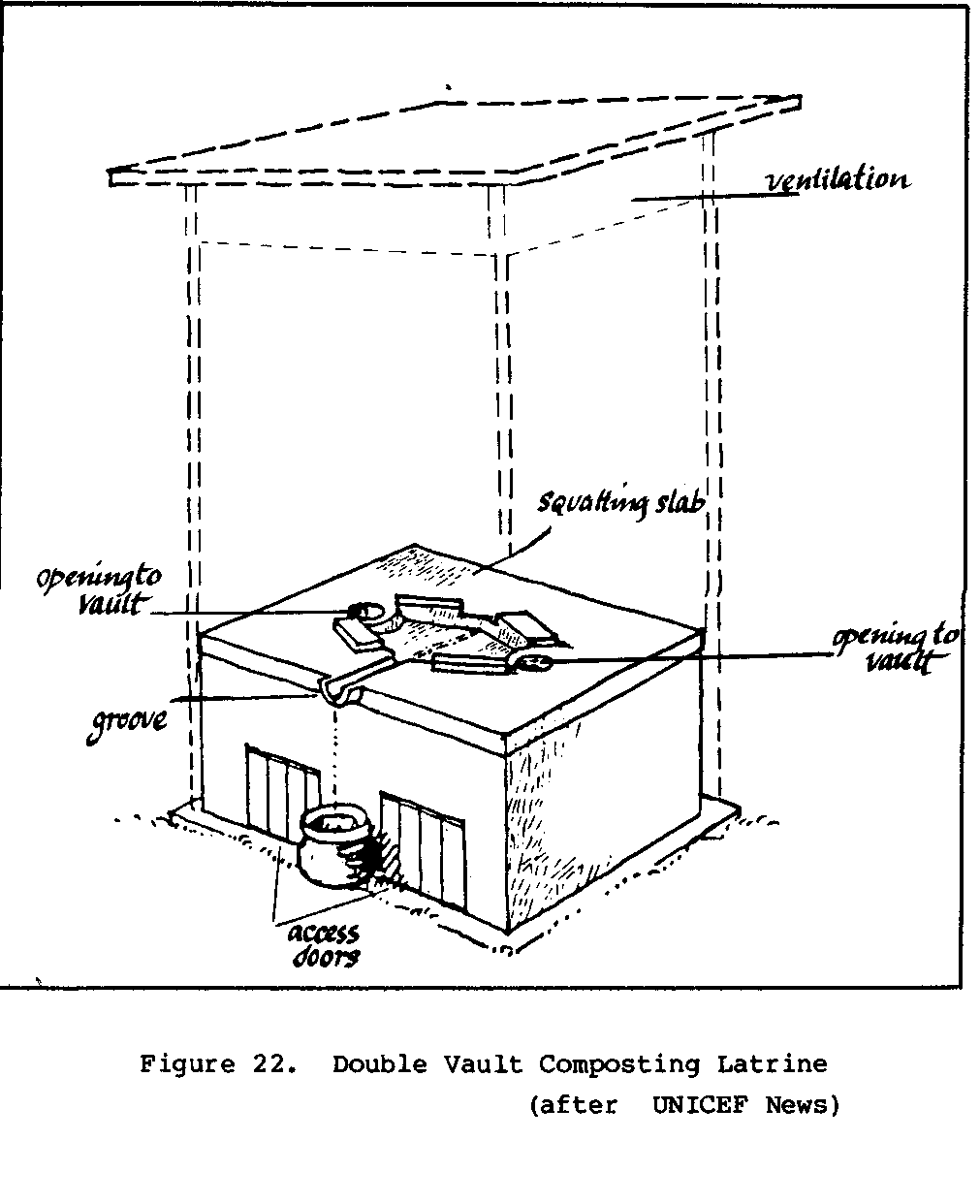
was developed for dry composting of human excreta. Two
cement-lined vaults are constructed above ground level
with access doors for each vault. The vaults are covered
with "squatting plates", one opening into each
vault. One
vault is used until filled, then sealed off while the
other vault is in use. After 45 days, the filled vault is
opened and a rich, harmless, odorless fertilizer is removed
through the access door. Urine is separated from
fecal matter by a groove in the squatting slab. The latrine
design is being promoted by UNICEF in other Asian
countries.
Wet composting methods offer equally sound alternatives
for waste disposal and are becoming increasingly common in
developed and developing countries. The People's Republic
of China has reported success with a three-stage method of
waste disposal that is particularly effective in reducing
the incidence of schistosomiasis. In this design, a
container
with three internal compartments is used to render
the wastes less hazardous to human health and produce a
valuable by-product (Figure 23). Wastes are introduced
esw23x79.gif (486x486)

into the first compartment through a water trap. The
wastes begin to undergo anaerobic decomposition and the
schistosome eggs, being heavier than water, begin to
sink. The diluted excreta moves into the second compartment
while decomposition continues. When the material
reaches the third compartment, decomposition may be nearly
complete and all of the schistosome eggs have been removed
or rendered inactive by chemical changes in the effluent.
The residues removed from the third compartment are a
valued resource to be spread on cropland or added to fish
culture ponds.
Initial cost is high, as in dry composting, and the residue
is likely to contain pathogens other than schistosomes.
Anaerobic decomposition proceeds at a much slower
rate than aerobic decomposition and does not generate as
much heat; therefore pathogenic bacteria will survive the
process. Anaerobic decomposition is also likely to create
a thick scum on the surface which hardens enough to require
a complete clean-out on a regular basis.
Another method of waste disposal exploits another feature
of anaerobic decomposition. When the bacteria break down
wastes without oxygen, a gas containing methane is produced.
This biogas is combustible and produces between
30-60% of the energy contained in natural gas. Large and
small biogas plants have been built around the world to
supplement dwindling supplies of firewood or petroleum
products. It is estimated that there are over 10,000
plants in operation in India, 29,000 in Korea, 7,000 in
Taiwan and 80,000 in the People's Republic of China.
The amount of gas produced from wastes depends upon several
conditions, the most important ones being temperature
and the type of waste used. For example, the dung from
one medium-sized animal (cow, bullock or buffalo) can
produce 500 to 600 liters of gas per day while the daily
production of gas from a human's waste is only 30 liters.
The optimum temperature is either between a low range of
30-40 [degrees] Celsius (C) or between a high range of 48-60
[degrees] C.
The farther away from either temperature range, the greater
the reduction in gas production. The higher range
(48-60 [degrees] C) is usually not considered practical for
small-scale
projects as it requires supplemental heat.
Peter-John Meynell's book, Methane: Planning a Digestor,
has all of the elements for planning and designing a
biogas plant.
While it is beyond the scope of this book to describe the
various types of biogas producers or anaerobic digestors,
a simple design can be offered for small-scale use (Figure
24).
esw24x82.gif (600x600)
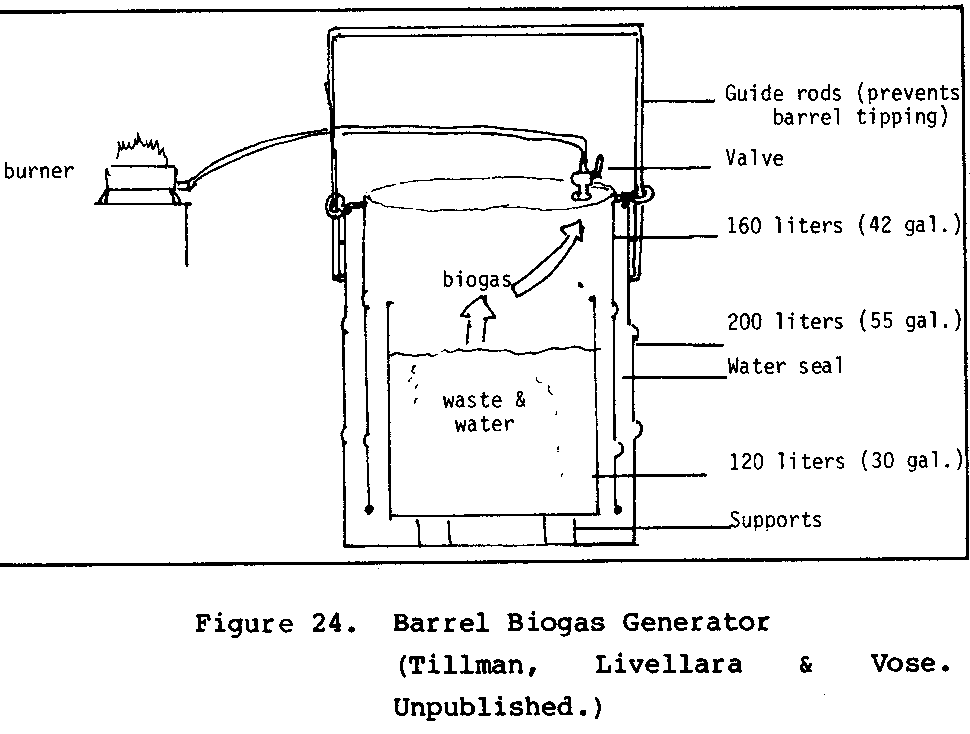
This digestor uses three barrels (200 liters, 160
liters and 120 liters) without tops commonly used for
chemical
or petroleum products, some metal tubing or rods, a
simple valve and some flexible tubing (rubber or
polyethelene).
The smallest barrel is placed inside the largest
barrel and filled with wastes (dung, sawdust, leaves,
kitchen wastes, urine) and partially filled with water and
the middle barrel is inverted over the smallest barrel.
Water is added to the larger barrel to make an airtight
seal. The rods keep the barrel from tipping as it rises
from the pressure of the biogas bubbling out of the
wastes.
The digestors are odorless as is the gas, which can be
burned without further processing. The holes on the
burners may have to be enlarged if the burner was designed
for bottled or natural gas. It would be wise to spill a
small amount of oil on the water in the larger barrel to
eliminate mosquito larva.
After the gas begins to give out, usually between 20-28
days, the waste materials must be replaced. With each
filling, there is a delay in gas production for 2-3 days
until the bacteria become well-established. What happens
to the residue after digestion? It is an excellent soil
conditioner and can be added to the garden, fish culture
pond, or dried and fed to livestock.
While not completely pathogen free, all the schistosome
ova will have become inactive. Biogas digestors need more
refinement but, even in a primitive state, they take
community wastes and return two very useful products.
Microbial decomposition systems, or digestors, do offer
great potential for the developing countries.
Experimentation
with these units on a village or multi-family level
will improve the technology and reduce the costs of
construction.
Wider practice of controlled decomposition can
relieve energy shortages, return valuable nutrients to the
soil, improve water retention capacities of porous soils,
reduce ground and surface water pollution, and lower the
incidence of water-related disease. The higher initial
costs are well worth the long range multiple benefits,
environmentally and economically.
6. WATER
AND AGRICULTURE <see image>
esw6x83.gif (393x393)
"And we created from water
every living thing."
The Koran
The role of water in agriculture is well-known, especially
by farmers in arid and semi-arid regions of the world.
Good crop growth depends upon the availability of plant
nutrients in the soil, sunlight and adequate water for
seed germination, growth, flowering and maturation. For
the most part, developing countries have plenty of sunlight
and have undeveloped areas of potentially arable
land. In the wetter parts of the tropics, rain-fed
cultivation
is the common practice. In these areas, the limiting
factor in crop productivity is soil fertility. The
farmer must control the movement of water so as to minimize
the loss of soil nutrients and changes in soil
structure.
In arid or semi-arid regions, there is little doubt that
water is the limiting factor and farmers must select crops
with low water requirements, develop techniques to conserve
water, and devise methods to provide water other
than rainfall to the crops. As a broad generalization,
soils in arid and semi-arid regions tend to be more fertile
than soils in the moist tropics. The except ions
would be the rich alluvial soils in the flood plains of
large tropical river systems. These soils are fertile
whether in moist or dry tropics.
Historically, the type of agriculture practiced in the
tropics reflected the nature of the environment. In the
moist tropics, farmers developed the "cut and
burn" or
"swidden" cultivation, where patches of forest
were cut,
burned and sown to crops. The new plants profited from
the release of nutrients from the ashes for 2-3 years.
When the nutrients were depleted, the farmer moved to a
new site and repeated the cut-burn-sow process, allowing
the abandoned plot to capture nutrients and slowly return
to forest. The system, still widely employed in the moist
tropics, does provide ample crops if human population
densities
are low and the abandoned plots have sufficient
time to return to forest before being cropped again.
Increasing agricultural pressures and changing patterns of
land ownership have shortened the fallow period (time of
natural regrowth) so that there is not enough time to
replenish the nutrients necessary for a healthy crop. In
addition, as more and more new forest is cut for agriculture,
the hidden environmental services performed by natural
forests are lost. Natural forests, besides accumulating
and storing nutrients, protect soil, retain water (in
plants and soil), provide habitat for wild animals and
native plants, give haven to aboriginal populations, purify
air, moderate micro-climate and maintain a repository
for the genetic information and characteristics contained
within the thousands of plant and animal species, some
found only in moist forest and some yet to be discovered
by science. The rate of moist forest removal is cause for
world-wide alarm and agricultural practices must therefore
be modified so as to get maximum production from each
hectare cut, if forests are to survive.
In the dry tropics, the agricultural patterns were
different,
but with the same basic concept of fallow agriculture.
The lands were often occupied by nomadic tribesmen
who would move livestock from area to area to capitalize
on small seasonal water supplies and forage. In the less
arid regions, some farming would develop with a pattern of
crop and fallow, but in this case it was in order to benefit
from the moisture which would accumulate in the soil
beneath the native, fallow vegetation. If the fallow
period was long enough, soil moisture would increase to a
point where one crop could be sustained over a growing
season. As in the moist tropics, conditions have changed
due to population increases and land tenure pressures,
resulting in shorter, inadequate fallow periods. To meet
agricultural needs in the dry regions, irrigation has been
widely introduced. It provides obvious benefits, but also
can create many perplexing environmental and social
problems.
Many of the problems of tropical agriculture stem from the
misapplication of agricultural technology. Agriculturalists
from developed nations assumed that the technologies
which produced the massive crop surpluses in temperate
zones could be transferred to tropical soils and climates.
To a distressing extent, the results of this
transfer have been disastrous, economically, socially and
environmentally. Although some are still attempting the
transfer, we have generally come to realize that temperate
agricultural methods are not likely to be successful in
tropical developing countries. Despite its recent origins,
research in tropical agriculture has produced exciting
results. Among other things it has given direction to
environmentally sound water development for agriculture.
WATER AS TRANSPORT
A quick review of the water-agriculture relationships will
provide a common base for discussing environmentally sound
projects. For good or ill and of ten both, water moves
things on agricultural lands and within plants.
Regardless of the sources, water moves matter to and from
agricultural sites, chemically and physically.
Chemical Transport
Many minerals, nutrients, pesticides and other chemicals
are dissolved and carried in water through surface runoff,
subsurface flow or percolation.
As overland flow, water moves by gravity toward streams,
picking up chemicals, nutrients and soil particles on the
way. Depending upon the amount of runoff, the kinds of
materials carried and the amount of materials dissolved in
the water, a number of negative effects can result from
chemical transport. Increased nutrients can promote
uncontrolled growth of algae and waterweeds in water
bodies. These plants are capable of cutting off sunlight
to the bottom plants, reducing their capacity for
photosynthesis. As the algae and waterweeds die, decay
depletes the oxygen in the water which, when coupled with
the lower rate of photosynthesis, kills fish and other
aquatic animals. Pesticides in runoff are also lethal to
aquatic organisms at very low concentrations. Excessive
nitrates and phosphates from agricultural fertilizers are
health hazards when they are allowed to run off and
contaminate domestic water supplies.
Percolation may move nutrients down below the root zone of
plants where they are useless to agriculture. This is the
major reason for the infertility of tropical soils in
areas that receive over 2000 mm of rainfall annually. The
amount and frequency of deep percolation depend upon the
soil structure, amount of organic materials in the soil,
the vegetative cover (amount and type), amount of rainfall
and the underlying geologic formation.
Percolation has beneficial effects as well. Percolation
is necessary for the recharging of ground water. Another
benefit is the moving of dissolved salts deeper into the
soil where they are not injurious to plants. A large
percentage of the arid soils contain high levels of salt
and percolation is necessary to remove those salts before
crops are planted.
Physical Transport
Raindrops falling on unprotected soil strike with amazing
force, adequate to dislodge particles and carry them from
the site. Within limits, this movement--or erosion--is a
natural and necessary process within the ecosystem. A
flow of nutrients to aquatic environments is needed to
maintain biological systems. The rich valley and plains
soils are derived from erosion of an earlier geologic era
and the fertility of flood plains is enhanced by the annual
increments from upstream highlands. Erosion, then,
is an important part of natural ecosystems, but can have
unpleasant results when it is allowed, or encouraged, by
man to become excessive.
Water carrying the suspended soil particles eventually
slows when the topography becomes less steep. As velocity
decreases, the heavier particles settle as stream or lake
sediments, which can choke or divert streams, increase
flood potential, foul turbines or cooling devices, and
kill aquatic life. The suspended lighter material not
only decreases passage of sunlight, but also presents a
filtering problem for domestic or commercial water supplies.
Unfortunately, some development programs have failed to
institute necessary erosion control measures and have
actually increased the rate of erosion, thereby further
impoverishing the people who were to benefit. In nearly
every case, closer examination has shown that increased
erosion was an unneccessary cost which could have been
avoided through careful planning and little additional
expense.
SOURCES OF WATER
Those seeking to develop agricultural water sources can
generally be guided by the discussion of water sources in
Chapter 4. There are some differences worth noting,
however, since they do influence project planning. For
instance, although it should not be assumed any water
source can safely be used for cultivation, agricultural
water supplies do not have to meet as high standards as
those for drinking water. The presence of pesticides,
nutrients and high bacterial or algal populations does not
necessarily compromise use of water for agriculture. In
fact, the additives may be beneficial to crop production.
Unlike domestic water requirements, agricultural needs are
seasonal, limited to all or parts of a growing season.
Normally, small-scale projects need not provide water for
year-round irrigation. Crops can be selected to take full
advantage of a wet season and supplemental sources may be
needed only for short periods at the beginning or end of
the growing season.
Water resources for agriculture can be intermittent.
Unlike humans, or industry, most crop plants do not
require a constant flow or constant volume of water.
Plant water requirements vary with time in the life cycle
(i.e., germination, growth, flowering, maturation) and
during some stages plants are capable of surviving with
little or no rainfall. Using the soil as a water reservoir,
plants can endure long periods between rainfalls or
irrigations.
Because of these differences between agricultural and
other uses, the planner has a wider range of sources to
tap for development. For instance, a surface source
unacceptable
for human consumption because of algal blooms or
fecal contamination can still be used for irrigation. On
the other hand, water may contain levels of boron, for
example, which are not injurious to humans but are toxic
to plants. Or, more common in arid lands, water may contain
salts which affect taste but are not necessarily
harmful to humans, yet can accumulate in the soil and kill
plants. So, while issues of water supply and quality are
less demanding for agriculture, there are still important
limitations.
UTILIZING WATER RESOURCES
Since rainfall cannot be increased except by unreliable
and expensive technology, ways must be found to increase
the agricultural utility of the amount that does fall.
There are several possibilities that may produce excellent
results.
Increased Water Retention
Maintaining a vegetative cover is the best method to
retain soil moisture. Surface runoff is greatest when
land is stripped of vegetation. In order to minimize this
loss, "no-tillage" agriculture is increasingly
used with
good results. In this method, the vegetation is not
burned nor plowed under in preparation for seeding. Small
areas are slightly opened to receive the new seeds and as
the crop begins to grow, competing weeds are removed by
hand. A plant canopy shields the soil from direct sun and
prevents high evaporation rates all year, first by weeds
and grasses, and later, by the growing crops. Water loss
and erosion are controlled by this technique.
When plowing is necessary, water can be held longer if
furrows follow the contour, cutting across slopes instead
of up and down the slope. In this way, furrows act as
barriers to the flow of surface water. Unplowed strips
also accumulate soil moisture for the next season's crop.
If the unplowed strips consist of legumes (a large plant
family which includes alfalfa, clover, beans and
groundnuts),
the soil will be enriched with nitrogen and a crop
can be harvested. World Neighbors publishes inexpensive
and easily understood directions for making contours (see
Appendix I). Terraces are another means of halting surface
runoff, but involve more careful design and construction
skills than do the other options.
Increasing the organic content of soil aids in water
retention. By adding humus or the residues of composting
to the soil, more water is held between the soil particles.
For best results, the humus or compost should be
worked lightly into the soil.
Organic mulching, or covering soil with agricultural
wastes, is a proven method for retaining water and reducing
erosion. Stalks, leaves and inedible parts of crops
and other decaying material spread over the surface of the
soil reduce evaporation and provide a physical barrier
against wind and water erosion, while releasing nutrients
to the soil.
Crop Selection
In most areas, crops are selected for reasons other than
their water efficiency. As a result, they use far more
water than other crops which could produce the same amount
of nutrients. Some cash crops transpire large amounts of
water during the growing season. By selecting crops with
lower transpiration rates such as barley, sorghum, millet
or beans, less water is lost from the small rain water
supply. Table 5 summarizes water requirements of some
plants.
Table 5. Plant Water Requirements
Low
Barley
Beans
Millet
Oilseeds
Onions
Potatoes
Sorghum
Tobacco
Moderate
Corn
Flax
Soybeans
Sugar beets
Sweet potatoes
Higher
Alfalfa
Citrus fruits
Cotton
Grapes
Rice
Sisal
Highest
Bananas
Cocoa
Coffee
Dates
Sugar cane
Since the amount of water used is governed in part by the
length of the growing season, planners can conserve water
by selecting crops that mature more rapidly and require
less water. Under optimal rainfall conditions, these
crops would have small yields than traditional or highyield
varieties. But during years of limited rainfall,
they will produce relatively higher yields.
Many crop varieties have been developed especially for
their water-saving characteristics. For instance, a
short-strawed wheat is used in arid lands because it
transpires less water than the varieties with taller
stalks. In many cases, researchers have developed strains
or varieties that do better in drier climates without
reductions in crop yield.
Crops can also be selected for their ability to thrive on
water of lower quality. The saline waters of some arid
regions cannot be used on crop varieties which are
successful
in temperate regions, but salt-tolerant strains
are available. Certain types of cotton, barley, wheatgrass,
sugar beets, olives, date palms and pistachios have
been cultivated successfully under conditions too saline
for other crops.
Another exciting and potentially rewarding possibility is
to cultivate plants which have not been traditionally
exploited for agriculture, but which thrive in a variety
of climates and soils. The National Academy of Sciences
publication, Underexploited Tropical Plants with Promising
Economic Value, describes 36 possible crops covering
cereals, roots and tubers, vegetables, fruits, oilseeds,
forage and other non-food crops. Some of the plants are
adapted for moist tropics, others for dry tropics and
still others for saline environments.
Timing of Planting
Much of the world still determines the time of planting by
other than scientific methods. Planting by "signs"
and
"spiritual messages" or predetermined planting
dates is
still very much with us. Fortunately, some of the planting
practices which appear to be based on traditional or
supernatural events are actually based on knowledge gained
through centuries of trial and error and cannot be improved
upon. In other cases, these non-scientific methods
miss the most opportune planting times. By selecting the
earliest planting date when temperatures are cooler and
soil moisture highest, the crops get maximum benefits from
reduced evaporation and residual soil moisture. The wise
planner will take a careful deliberate look at traditional
practices to see if they maximize moisture retention of
the soil.
IRRIGATED AGRICULTURE
Irrigated agriculture involves very complex soil-water
interrelationships and should not be entered into lightly
or in ignorance. Agricultural benefits can be high but
environmental repercussions may well reduce the
benefits--often
to the point where costs exceed benefits and
endure long after the project has ceased yielding any
positive returns.
Irrigation has been practiced for over 5000 years. Some
irrigation systems in Mesopotamia, Egypt, India, China and
Peru, dating back thousands of years, are still in
operation.
Today irrigation technology is rapidly expanding
through experimentation, and new knowledge permits the
introduction of irrigation on sub-marginal lands and on
smaller scales. However, the environmental consequences
of a poorly planned and managed system remain perilous.
Peter Stern's recent book, Small Scale Irrigation (see
Appendix I), is the most useful reference for anyone
planning
or implementing a small-scale irrigation project.
There is no single "best" method of irrigation. An
appropriate
method, always a compromise, will depend on several
factors, including:
*
supply and quality of water
*
distance between water source and land to be
irrigated
*
topography of site
*
infiltration and percolation rates of the
soil
*
water-holding capacity of the soil
*
chemical characteristics of the soil
*
moisture requirements of the crop
*
climate
*
amount of funds available
*
amount of skilled and unskilled labor
*
prevalence of water-related disease
*
experience with irrigated agriculture
*
cost of energy.
In spite of its length, this list is not exhaustive nor is
it arranged in order of priority, but it helps to
illustrate the complexity of irrigation decisions.
Irrigation projects can have far-reaching effects on the
environment over an area larger than the project site.
Irrigation has triggered dramatic increases in water-related
diseases, especially schistosomiasis and malaria.
Infection rates for schistosomiasis have jumped from 10%
to 80% of the local population in some new irrigation
areas. Insect pest populations, normally reduced to low
levels during dry seasons, prosper from the year-round
water, thereby increasing the reliance on expensive
pesticides
for control. Irrigation can also have an impact on
water table depth, water quality, soil productivity, as
well as consequences for society in terms of family
structure,
human mobility patterns, economic status of farmers
and land ownership patterns. Hostilities between neighbors,
adjoining communities and even countries have resulted
from disputes over irrigation water and practices.
Just as irrigation projects affect the environment, some
environmental factors can have a devastating impact on
irrigation. Deforestation or mismanagement of the watershed
can cripple an otherwise well-designed irrigation
system. Industrial wastes from upstream sources may kill
crops and render soils unsuitable for agriculture. Increased
soil salinity or water-logging may put croplands
out of production and be terribly costly to correct. Each
year, thousands of hectares of agricultural lands are lost
due to poor water control, often in small-scale projects.
Planners must take precautions to ensure that environmental
changes outside the project area will not have
uncontrollable
negative effects.
EFFECTS OF USING SURFACE WATER FOR IRRIGATION
Irrigation water from surface sources, usually diverted
via canals, ditches or closed conduits, is commonly used
for small-scale projects. The diversion of the water can
affect aquatic and terrestrial environments.
Aquatic Environments
*
Removal of water from rivers and streams
reduces
downstream
flows, decreasing habitat for aquatic
plants and
animals.
*
After irrigation, water returned to surface
sources
is of poorer
quality than original water, often
containing
substances lethal to aquatic organisms.
*
Lowered streams or rivers entering the sea
will
suffer
increased encroachment of saltwater.
*
Reduced water flows may cause increased
siltation
and
sedimentation of rivers downstream.
Terrestrial Environments
*
Irrigation may increase the amount of
subsurface
water until
the water table rises into the plant
root zone. The
raised water table inhibits the
growth of most
agricultural crops by water-logging
the soil, so
that the exchange of oxygen between
roots and soil
pores cannot take place.
*
The elevated water table may leave standing
water
at the surface
providing ideal breeding sites for
crop pests as
well as snail and mosquito vectors of
disease.
*
As evaporation from high water tables
increases,
salt residues
form unless the water is
exceptionally
free of salts. The salts may remain
at toxic
levels for a long period unless remedial
steps are
taken. Salinization caused by improper
irrigation is
the most common cause of abandoned
cropland in
arid or semi-arid regions.
EFFECTS OF USING GROUND WATER FOR IRRIGATION
The effects of using ground water for irrigation are
similar in many respects to the effects of using surface
water, especially in the terrestrial environment. If the
ground water is applied in excess and runs off to surface
waters, then the effects will be similar to those caused
by return flows from surface sources, although the volume
of the stream may be increased.
One significant difference does exist. Drawing irrigation
water from ground water supplies does lower the water
table, although not necessarily at the irrigated fields.
The lowered water table causes secondary effects which
must be considered by the planner:
*
Marshes, springs and seeps may dry up. These
areas
provide
habitat for wildlife.
*
Stream and river flows may be reduced.
*
Dug wells, used for domestic supplies, may
run dry.
*
Local vegetation, no longer able to reach
the water
table, may
die. Animals dependent on native plants
will disappear
or become crop pests. Plants which
are rare or
unique to the area may disappear.
MAJOR PROBLEMS IN IRRIGATION
The most frequent and major negative effects of irrigation
are water-logging, soil salinization, soil alkalinization
and increased disease.
Water-logging is caused by seepage from irrigation canals
or over-application of water, accompanied by insufficient
drainage. To correct this may require artificial drainage
systems--either open interceptor ditches placed at intervals
on the fields to collect and drain excess water from
the fields, or subsurface drains consisting of a series of
perforated tile, concrete or plastic pipes in the soil.
Subsurface drains, although more expensive, are the
preferred
option because they do not take up crop space,
require less maintenance and do not provide habitat for
disease vectors.
Farmers often operate on the philosophy that if a little
water is good, more is better; therefore, they over-irrigate
crops. One way to increase irrigation efficiency is
to levy water use fees. Even if charges are minimal,
farmers will be less likely to waste water. The fees can
be used to pay for the routine maintenance of the system.
Salinization is the accumulation of mineral salts--sodium,
calcium, magnesium or potassium--in the upper soil layers,
including the plant root zone. A white crust or powder on
the surface is characteristic of severe salinization,
although crop yields decline before crusting appears.
So-called secondary salinization occurs when ground water
rises, carrying the salts upward to the root zone, and
evaporation at the surface leaves the salts behind. If
fields are well-drained and sufficient water is applied,
the salts will generally leach down out of the plant root
zone and will not affect plant growth. It is unfortunate
that irrigation drainage does not receive the same degree
of attention as the development of the water supply.
Alkalinization is a less common but more serious problem
than salinization. Alkaline ground waters or sodium-rich
irrigation waters can increase sodium ions in the upper
layers of the soil by the same processes which cause
salinization.
The sodium ions change the soil structure,
making it difficult to till and nearly impermeable to
water. Alkalinization can be ameliorated by deep horizontal
drains, application of large doses of organic manures,
and permanent use of acids, but a cure requires expert
analysis and treatment.
Increased disease associated with irrigation and other
water projects has been treated earlier in Chapter 3.
IRRIGATION METHODS
The type of irrigation selected for a small-scale project
depends upon many variables; each method has its advantages.
Some have specific site, water or material requirements.
A brief summary of the different methods will
highlight some of the benefits or limitations of each
type.
Watering Can
The simplest form of irrigation is with a watering can.
With this technique, one man can manage a garden of about
500 sq. meters if the water source is adjacent to the
plot. If the water source is 500 meters distant, he can
manage only 250 sq. meters, illustrating the obvious
disadvantage
of this method.
Subsurface Clay Pots
Like the watering can, this method is labor intensive but
requires little capital investment. Crude, unglazed clay
pots are buried in the soil next to the crop seeds. The
pots are filled with water and covered with a lid. Moisture
slowly seeps through the sides of the pot in amounts
sufficient to sustain many crop plants. The pots are
refilled when necessary.
Pots can be made by unskilled labor, and children can help
manage the system. Since pots are covered, they will not
provide breeding sites for snails or mosquitoes. Water is
conserved because seepage will not exceed the plants'
requirements, and evaporation is minimal. There is no
threat of water-logging or salinization, and the technique
is well-adapted to no-tillage farming. It is possible to
substitute other materials for the clay pots (e.g., bamboo
tubes, gourds or cans with fibrous mesh covers) as long as
small openings can be made in the container.
Both of these methods are economical of water but are
labor intensive. They are best used with fruit or vegetable
crops where individual plants have high value and
are spaced so that moisture from the pots can reach each
root system.
Basin Irrigation
This method is widely used and easy to operate. The
basins, ranging in size from 1 sq. meter to several
hectares, are surrounded by low banks (30-50 cm) called
levees, bunds or dykes. The size of the basin depends on
land ownership, topography and soil characteristics.
Since the basins must be level, the amount of earth-moving
can be reduced if smaller basins are used rather than
large ones. Water is flooded into each basin from pipes
or ditches and is allowed to soak into the soil. Rice,
cotton, grain, maize, groundnuts, vegetables and orchard
trees can be irrigated by the basin method. In fact, if
each orchard tree has a basin, it doubles as a
micro-catchment,
collecting rainwater from a larger area.
Water input must be carefully regulated so as not to
saturate the plants' root zones for extended periods. For
crops other than rice, water should not be allowed to
stand for longer than 24 hours.
Basins are arranged adjacent to supply channels and water
is passed through gates (turn-outs) or siphons to each
basin, as required. Ideally, basins should be constructed
as narrow rectangles with the long axis perpendicular to
the supply channel, so that the largest number of farmers
can be served by a water supply channel of given length
(Figure 25).
Basin irrigation is not capital intensive and requires
very little equipment. Land leveling can be done by hand
or by draft animals pulling simple scrapers. Levees can
also be constructed and maintained by hand tools or with
simply fabricated ridgers.
Basin irrigation does pose health hazards, providing
breeding sites for snails and mosquitoes. Water-logging
and salinization are also threats unless drainage is
provided.
The levees can be destroyed by draft animals or
wild animals. Erosion is not a serious problem.
Border Irrigation
This method makes use of parallel earth levees (20-25 cm
high) to guide water down a long gentle slope, thereby
irrigating the land between the levees (Figure 26). The
esw26x10.gif (437x437)
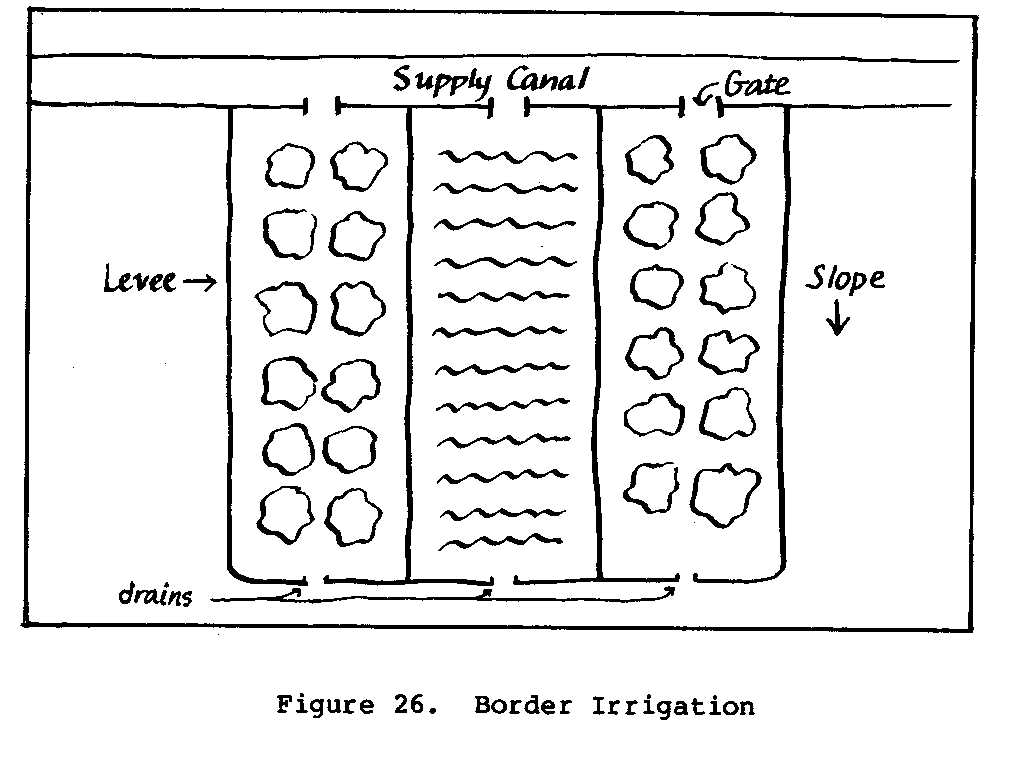
width of the border (3-30 meters) and length (100-800
meters) depend on water supply, soil permeability and
degree of slope. Periodically, a large volume of water is
gated in and a sheet of water proceeds down the slope,
percolating into the ground. If the proper amount of
water is applied, all the water will have infiltrated into
the soil as the leading edge of the water reaches the end
of the border, and the risk of water-logging, salinization
and disease will be small. Drains at the lower end will
draw off excess water. Success with the system depends on
an understanding of the relationship between slope and
rate of infiltration, so it is not well-suited for
small-scale
projects.
Wild Flooding
This method consists of spilling water through gates or
siphons from a canal along the upper border of a sloping
field. Water is allowed to flow freely down the field
unless diverted by interception ditches or bunds. The
method is best adapted for pasture or forage crops, where
exposed soil is minimal. Efficiency is low, as the water
released is not precisely controlled.
Furrow Irrigation
This method involves moving water in small channels
(furrows) between rows of crops, down a gentle slope to a
drainage ditch. Frequently used for high-value vegetable
or fruit crops, it is the most complex of open-channel
irrigation methods. Precise land grading is necessary and
the furrows require continued attention. Furrow irrigation
can produce excellent results and can be adapted to
small-scale ventures. In large systems, the canals are
often deep and water is controlled by gates. In small
systems, supply canals can be dug by hand and water diverted
by shoveled openings for each furrow. The length of
the furrow can be determined by experience, since it will
depend on water flow and soil porosity. For small-scale
projects, the method is best used for higher value crops
which cannot tolerate variable rainfall conditions.
Sprinkler Irrigation
This method of irrigation is widely used in the developed
nations but is normally inappropriate for small-scale
projects.
Water is sprayed on or beneath crops from high
pressure sprinklers. The materials, usually imported, are
expensive and require frequent replacement. The system is
highly efficient and eliminates most health hazards.
Since water volumes can be precisely regulated, waterlogging
and salinization are unlikely. Unfortunately,
this capital intensive method is not likely to be employed
for rural small-scale projects.
Trickle Irrigation
Also known as drip irrigation, this is a high-technology
version of the clay pots and is costly to install and
maintain. In this method, hoses or pipelines deliver
small amounts of water to the bases of high-value plants
as needed. The tiny openings in the pipe clog easily so
irrigation water must be filtered in advance. It is possible
that local materials such as bamboo can be used;
otherwise the cost will be prohibitive, which is
unfortunate,
because trickle irrigation can be very efficient and
avoids the environmental problems of other irrigation
systems.
Author's note:
Before embarking on an irrigation project,
the reader is
urged to secure a copy of Peter
Stern's Small
Scale Irrigation. It is a most useful
source of
information.
7. AQUATIC
PRODUCTS <see image>
esw7x107.gif (256x256)
"And he has set up the
balance in order that ye
may
not transgress
balance."
The Koran
Harvest of aquatic products can provide important
supplements
to the nutrition or the economy of local areas.
Culture projects with fast-growing fish species have often
been highly successful and have great potential for
protein-deficient
countries. Annual fish harvests exceeding
1000 kg per hectare have been demonstrated in several
countries and the technology is suited for other areas.
However, the success of artificial culture techniques such
as fish ponds should not obscure other alternatives, such
as the harvest of natural plant or animal products and for
uses other than nutrition. Many plants or animals can be
exploited on a sustained-yield basis to provide raw
materials
for craftwork, such as jewelry, clothing or furniture.
If the plant or animal can provide food as well, so
much the better. Obviously if an aqua-culture project
depends on fresh water, it will be more appropriate to the
moist tropics than to arid areas.
ENVIRONMENTAL GUIDELINES FOR NATURAL PRODUCT PROJECTS
*
Regardless of economic value, recognized
rare or
endangered
species should not be harvested. In
spite of
increasing international limitations,
trade in
endangered species, for pets or hides, is
still common.
Unfortunately, as species become
rare, the
economic value of the species increases,
encouraging
illegal trade. Development workers
must discourage
this type of activity.
*
Projects should be developed for sustained
yield.
The removal
rate of the plant or animal must not
exceed the
natural reproductive rate of the
resource.
*
The project must not jeopardize the existence
of
animals higher
in the food chain. If a plant or
animal is a
major food source for larger animals,
it should not
be removed to such an extent that
other animals
in the ecosystem will suffer.
TYPES OF NATURAL PRODUCTS FOR SMALL PROJECTS
The harvest of native fish is generally well-established,
although in many areas certain species are not taken
because there is no market for them. A development worker
may usefully examine the operation of native fishermen to
determine if some types of fish are routinely cast away
and whether it is possible that new methods of processing
or preparation, such as fish meal, can win acceptance and
add to the community's protein supply.
Shellfish are often ignored as a food source, merely
because the community has little or no experience with
preparation or utilization. Clams, mussels or even snails
are excellent food sources and shells can be used to
fashion jewelry or souvenir items for sale in urban
areas. A warning here, however: shellfish are notorious
accumulators of toxic substances, including bacterial and
viral sources of disease. The water supply must not be
contaminated if the shellfish are to be used as food.
Native plants can also be used for foods or craft materials.
Plants normally thought of as weeds often produce
leaves, tubers or seeds than can be very nutritious. Care
must be taken to determine if any poisonous compounds are
present, although most toxic plants are rendered harmless
through cooking.
Seeds from native plants have been widely used for
decorative
items or jewelry. Fibers in the leaves or stalks can
be used for furniture, household goods or cords, as with
hemp. Other plant products may include resins for fuel,
dyes or adhesives. Native plants, including some serious
nuisances such as waterweeds choking waterways, can be
used for agricultural mulching, bedding for livestock or
as fodder. Insecticidal characteristics of some plants
can reduce insect infestations of the animals, if the
plants are used for livestock bedding.
AQUATIC CULTURE PROJECTS
Well-planned aqua-culture projects to produce fish,
seaweeds,
turtles or shellfish can yield many community benefits
without adversely affecting environmental quality.
The ancient practice of fish culture is more common than
turtle or shellfish culture and can be used as a model for
discussion.
The most popular fish varieties under culture are carp and
Tilapia, an African fish. The popularity of carp is due
to its rapid growth rate and high productivity in managed
ponds fertilized with night soil (human excreta). The
most commonly stocked carp include silver carp
(Hypophthalamichthys
molitrix) and big-head carp (Amstichthys nobilis)
which feed on plankton, common carp (Cyprinus carpio)
and mud carp (Corrhina molitcrella) which feed on bottom
detritus, and the grass carp (Ctenopharyngodon idella)
which feeds on pond weeds. The Chinese often mix these
species to maximize the production from the different
nutrient sources in the pond.
The Tilapias are prolific producers with possible yields
over 2000 kg/ha in fertilized ponds. The most common
Tilapias (renamed Sarotheroden sp.) for fish culture are
S. mossambicus and S. niloticus which feed on algae.
In preparing for a fish culture project, several environmental
factors must be considered, first being the amount
of water available for the ponds. Water supplies must be
adequate to fill the ponds and replenish water lost by
evaporation or seepage. Water must be free of toxic
chemicals
and, if unlined ponds are planned, the soil must be
relatively impermeable to water.
To avoid extensive leveling or earth-moving, the topography
of the pond site must be considered. To avoid pumping
it is important that the water source be higher than the
proposed pond or ponds. Accumulated bottom sediments and
organic debris should not be released into water courses,
where they will be polluting; instead they should be
removed and deposited on agricultural lands (if they are
nearby), where they will add valuable nutrients.
Fish culture can also be practiced in natural water
courses. In Indonesia, cages (karambas) are constructed
with 1-4 cm spacing between bars. The cages are stocked
with small carp and anchored in rivers. After 2-3 months,
the fish double in weight and 5075 kg of fish can be taken
from a karamba with a volume of only one cubic meter.
This type of culture is appropriate for nutrient-rich
rivers and estuaries.
The danger of human disease is high in most fish culture
projects, especially where ponds are fertilized with night
soil. The threat is increased if raw or partially cooked
fish are traditionally consumed by the villagers. The
incidence of liver fluke (Clonorchis sinensis) and lung
fluke (Paragonimus) and fish tapeworm (Diphyllobothrium
sp.) is increased, as each of these parasites requires
fish for the infective larvae. Cooking or drying will
destroy most of the parasites in the fish, but does not
reduce the levels of schistosomiasis or malaria associated
with aqua-culture.
A more complete and technical discussion of fish culture
can be found in Freshwater Fish Pond Culture and Management
by Marilyn Chakroff (see Appendix I).
8. WATER
AND ENERGY <see image>
esw8x112.gif (393x393)
"Fools rush in where
angels fear to tread."
Anonymous
The development of inexpensive, decentralized energy from
renewable resources is beginning to attract considerable
attention for small-scale projects, and rightfully so.
Recent advances in simple solar-powered devices, windmills
and biogas generators are encouraging for small-scale
use. It is only natural that water flow also be
considered an inexpensive power source. Unfortunately,
water-powered devices are not as sound as the other
alternatives.
Water can be used to power a pump, as with the hydraulic
ram. The water ram is a simple and inexpensive device for
pumping water higher than the original source, if topography
allows for a free-fall between the original source and
the pump. The water ram cannot pump all the water that
falls since more than 80% of the falling water is used for
pumping energy and less than 20% is actually pumped to a
higher elevation. For this reason, the water ram is only
useful in areas of abundant water or in situations where
the water is falling for other purposes.
For centuries water has been used to turn water wheels.
The designs have not changed appreciably over time and
furthermore have not been adapted to the wide fluctuations
in flow found in tropical water courses. The wheels are
usually not sturdy enough to withstand the turbulent
floods during the wet season and they may not have enough
water to operate during the dry season. Flumes or canals
which could direct part of the river flows to a water
wheel carry all the negative impacts of stream diversion
and are very expensive.
Low head hydroelectric power is often mentioned as
potentially
small-scale, but it is small only in relation to
the giant projects which cost millions of dollars. The
cost of low head hydroelectric turbines, transformers and
distribution facilities is still beyond the scope of
projects
discussed in this manual.
Any water power project in the tropics will experience
development problems. In arid regions water is usually
too limited for small-scale power projects. In the moist
tropics, the violent flows are difficult to harness at low
cost. With the rapid expansion of rural electrification
programs in developing countries, villages may do better
to press for expansion of electrical distribution from the
central government and concentrate their water development
efforts in other sectors, such as water treatment and
sanitization. Further, unless the community has had
experience with water-powered machinery, it is difficult
to gain community support for these types of projects.
Water-powered machinery also requires constant and skilled
maintenance. In most cases, the small amount of energy
derived from primitive water power devices is not worth
the effort, funds or materials expended, nor is the energy
in a form that is most useful, such as heat or electricity.
For small-scale energy projects, the development
worker is best advised to look toward more promising
sources of energy, such as solar and wind power or biogas
production.
9.
PLANNING (1) <see image>
esw9x115.gif (230x285)
"Why
care for people? Because people are
the primary
and ultimate source of any
wealth
whatsoever. If they are left out,
if they are
pushed around by self-styled
experts and
high-handed planners, then
nothing can
ever yield real fruit."
E. F. Schumacher
Planning as it is used in this manual refers to the process
of thinking through a water development project in
terms of all its components and how they interrelate. It
includes determining objectives, selecting strategies to
fulfill them on a sustained basis, evaluating costs and
benefits (including environmental costs), and developing
means to protect the project well into the future.
________________
(1) Adapted from "The Planning Framework" by
Laurel Druben,
Environmentally Sound Small Scale Agricultural
Projects, 1979.
It is not the purpose of this chapter to replace the many
available methodologies for planning projects, but rather
to offer a planning process which emphasizes environmentally
sound approaches to water projects. Experienced
development planners will not have to be convinced of the
need for some of the steps outlined here, although some of
the material relating specifically to water projects may
be of interest and incorporated into other methodologies.
The planning process is described for the benefit of those
planners who want a review of the total process.
Sound planning of small-scale water development projects
does not necessarily take a long time. It is recognized
that in many cases the projects are needed to meet a
serious crisis requiring immediate solutions and that some
environmental risks may be inescapable. However, even
short-term objectives can be more successfully met if the
planner is aware of some very basic planning methods and
knows the right questions to ask.
A PLANNING PROCESS
A sound and flexible planning procedure is shown in Figure
27.
Using the diagram as an overview of the planning
process, we can examine the components in more detail as
they apply to water development projects.
GUIDELINES FOR ENVIRONMENTALLY SOUND, SUSTAINABLE,
SMALL-SCALE
WATER PROJECTS
Setting guidelines is the first planning step. These
guidelines provide standards for design and evaluation of
alternative project options. Guidelines can be developed
for environmental, social, economic and cultural
considerations.
Since this manual is primarily concerned with
environmental issues, the guidelines for ecologically
sustainable projects will receive more attention. Sample
environmental guidelines require that projects:
*
maintain or enhance water quality
*
use water efficiently
*
protect native plant and animal populations
*
maintain or improve soil productivity
*
protect water rights of existing users
*
use existing social organizations and
cultural
values for
environmental rehabilitation and conservation
*
include plans for protection of native
species and
undisturbed
wild areas
*
maintain or decrease existing levels of
water-borne
disease
*
insure long-term sustainability of water
resources.
More specific ecological guidelines, such as maintenance
of an endangered species or unique habitat, may be added
if necessary.
In addition to the environmental criteria, the development
worker may wish to use criteria or guidelines which
reflect the principles of appropriate technology/appropriate
development. The project should:
*
make optimal use of locally available
material and
human
resources
*
have community support and involvement
*
be based on community-identified and/or
community-realized
needs
*
increase potential for community
self-reliance in
both short and
long-term
*
be compatible with available funding
*
make use of and adapt traditional
technologies
*
have reasonable time frame for the community
to
take responsibility
for the project
*
have potential for being maintained and
monitored
by the
community.
These guidelines are not definitive. They should be
adapted and expanded to specific projects by the development
planner.
Other guidelines may also reflect goals of a sponsoring
agency or financial constraints. Obviously, the project
will be governed by cultural guidelines developed from a
community profile and discussions with community leaders.
After the guidelines have been agreed upon, they should
not be changed during the planning process except for very
good reasons. The planners must adapt project options to
meet the criteria rather than altering the criteria to
satisfy project designs.
COMMUNITY INVOLVEMENT
Small-scale development projects should originate from a
community problem or perceived community need. It is
unpardonable to plan for a community instead of with the
community. Countless examples from all development sectors
can be cited to emphasize the importance of community
involvement in project planning and implementation. Planners
must realize that people, not water, are the most
important factors in water development planning.
A prerequisite for effective planning is continual
interaction
with the community. Without community involvement
a project will not necessarily be supported by the
people. Such a project may yield short-term benefits but
will ultimately fail. Community involvement is the only
base from which sound project planning can proceed.
COLLECT INFORMATION
Community participation should begin at the earliest
stages in project planning when the development worker
begins to collect information. The information collected
should yield: a community profile and an inventory of the
physical environment.
Community leaders can help the development worker with the
preparation of the community profile. This profile is an
extremely important planning tool if it is structured to
provide easy-to-use data on economic, social and cultural
characteristics of the community. Data can be added or
refined throughout the project development process. The
profile can include many topics, but the minimum profile
for water development projects should identify the
following:
*
social structure and family relationships of
the
community
*
cultural traditions and characteristic
behavioral
patterns of
the community
*
official community leaders and other people
of
influence
*
social or special interest groups and their
role in
community
decision-making
*
relative economic level of the community,
not
necessarily in
per capita monetary units, but in
relation to
other communities in the area
*
land tenure policies and practices
*
educational organizations including informal
methods
and extension
services
*
judicial procedures used to settle community
disputes
*
available health data including disease
surveys,
health
facilities and medical personnel
*
water management or water rights policies,
which
may appear to
be indistinct or informal
*
human resources which could be available for
a
water
development project, such as the type and
amount of
skilled and unskilled labor which could
be spared from normal community activities.
In addition to the community profile, an inventory of the
physical environment is required. For a small-scale project,
the inventory need not turn into an extensive environmental
impact study. It should be a quick assessment
of the natural resources within the project area. As the
project alternatives become more clearly defined, detailed
environmental data may have to be collected. However, the
preliminary information should include the following
topics:
Water
*
Location and size of local water sources
*
Users and uses of local water resources
*
Quality of water
*
Water delivery systems
*
Dependability of the water supply, annually
and
seasonally
*
Type of vegetation around water sources
*
Type of protection of water resources
*
Water resource extremes (flooding and
drought)
*
Type of watershed management or protection
Climate
*
Annual rainfall patterns (when and how much)
*
Annual temperatures
*
Amount of annual evapotranspiration
*
Pattern of winds (direction and velocities)
*
Intensity of solar radiation
*
Basic relative humidity information
Soil
*
Basic soil composition data (gravel, sand,
clay)
*
Amount of organic material in soil
*
Type of vegetation cover on soil
*
Depth of soil to bedrock
*
Soil permeability (relative rates of
percolation)
*
Amount of local erosion
*
Amounts of local fertilizers used
Agricultural Practices
*
Types of crops grown
*
Amount of crops grown for local use
*
Food shortages or surpluses
*
Common pests (birds, rodents and insects)
*
Common pest control practices
*
Comparative crop yields (compared to
national
averages)
*
Comparative crop yields (different farmers
in
community)
*
Type of agriculture (rainfed, irrigation,
flood
recession)
*
Factors limiting increased production
*
Amount and type of livestock grazing and
migration
Natural Communities
*
Amount of natural forest
*
Amount of natural vegetation other than
forest
*
Direct threats to natural communities
*
Common wild animal populations
*
Potential rare or endangered species
*
Degree of protection for natural areas
Many other areas could be considered to evaluate specific
project proposals. Use the information in this manual to
select other inventory items.
As with many stages of planning, the development worker
may want some additional assistance in compiling the
physical environment inventory. In this case, the
development
worker could:
*
Seek advice from local residents. Their
knowledge
and
understanding of changes in environmental conditions
are not
usually available elsewhere. They
are a resource
too important to be overlooked.
*
Contact local universities, government
agencies and
local
representatives of international organizations.
Often they
have a great deal of pertinent
information on
local soils, climate, terrain and
plants and
animals native to the region. They may
have insights
and suggestions for other resources.
NEEDS IDENTIFICATION AND ASSESSMENT PROCESS
In the needs identification phase, background data are
reviewed for the perspective they yield on the local
community's
view of needs and priorities. Records kept of
interviews with local residents can provide particularly
good insights, but all of the material collected should be
reviewed.
A careful reading and weighing of background data during
the identification phase may indicate need for several
small-scale project activities. For example, community
members may voice strong concern over both the need for
erosion control and for improved sanitation; the inventory
of the natural environment may have indicated a need for
crop irrigation. If the need is "discovered" by
the
development worker but not voiced by community residents
(as in the case of crop irrigation), the development
worker must decide where that need fits into community
priorities, if at all. After the needs have been
identified, the assessment process follows.
A needs assessment can be undertaken in two steps. The
first is to look more closely at each identified need in
terms of the size of the effort required and the kinds of
resources which would be necessary to meet it. This can
be done by preparing a Needs Assessment Sheet, as shown on
eswx127.gif (600x600)
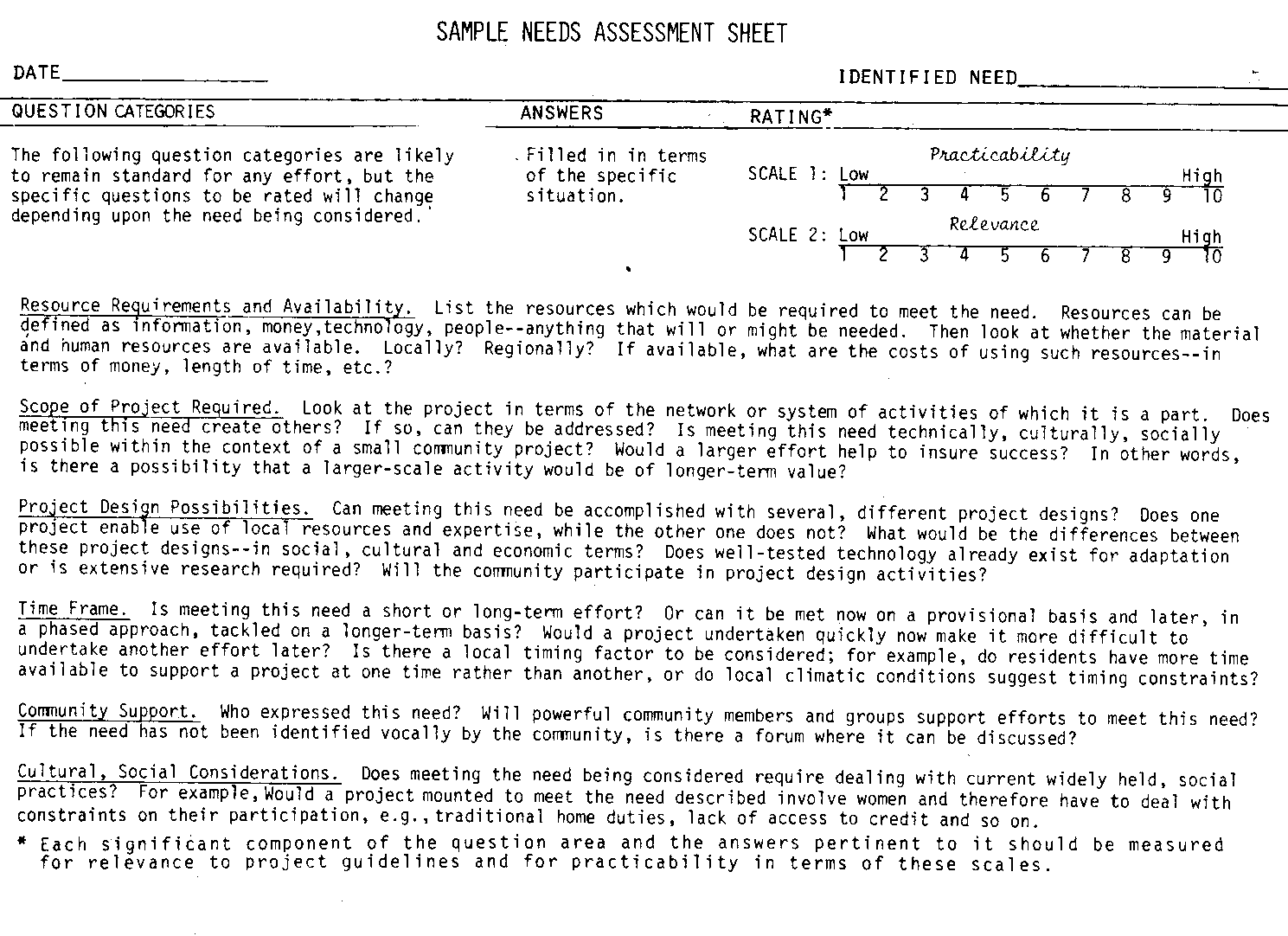
the next page, and by filling it out as indicated. Each
identified need should be placed on a separate sheet. For
each need it is necessary to define question areas which
have to be considered when preparing to work to meet that
need. The categories given on the sample sheet can be
refined or modified, based on the specific project.
Step two involves measuring the answers in terms of 1)
overall relevance to stated guidelines (page 118) and 2)
their practicability, given the type of effort required
and significant constraints which may exist, <see
scale> such as
eswx128.gif (600x600)
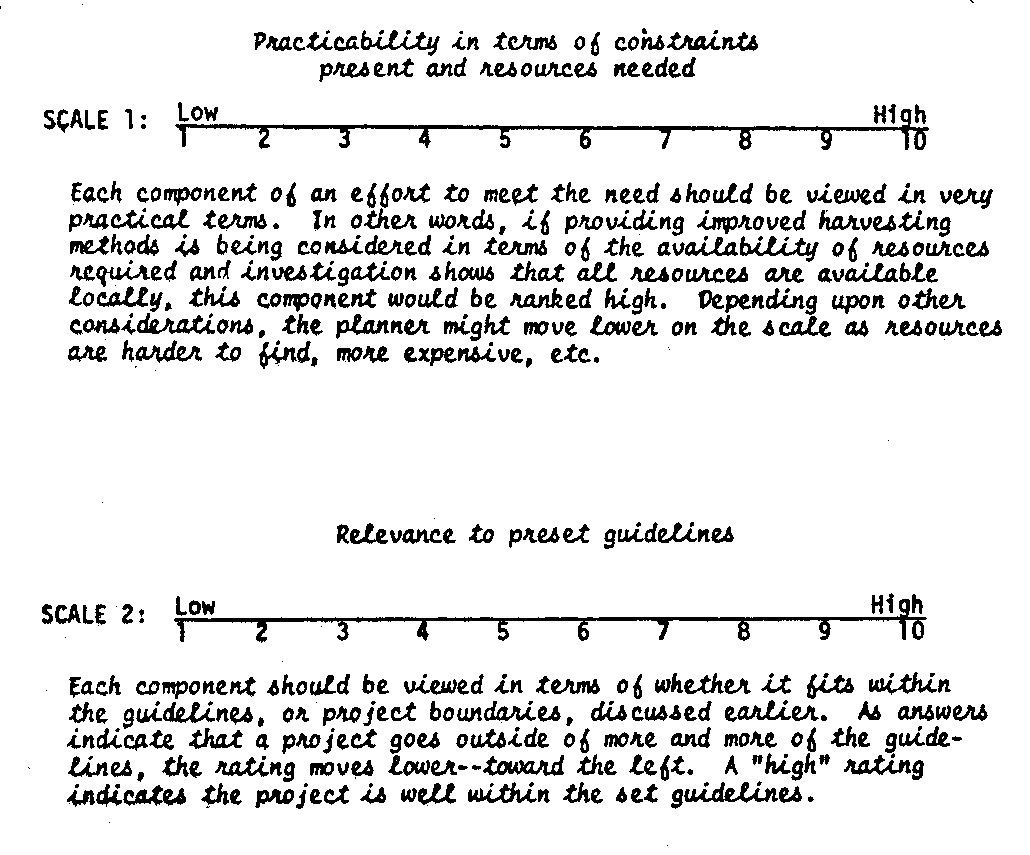
money, expertise or cultural bias. Two scales, numbered
from one to ten, should be set up. One scale is to measure
relevance; the other is to measure practicability.
Once each Needs Assessment Sheet has been completed and
the answers rated, average the two figures from the two
scales to determine an overall rating. A comparison of
the averages will give a fairly good indication of which
need is the one to tackle first.
PROJECT OBJECTIVES
The next step is to formulate project objectives which
reflect the priority need. These objectives must be
clearly defined: for instance, increase the water supply
so that each person will be able to have a daily allotment
of 38 liters of potable water. The project planners can
then accurately determine the dimensions of the task. A
goal of "improving the water supply' is basically
meaningless
because it does not define what is to be achieved. A
clearly defined objective not only sets the task precisely
but also provides a standard for measuring project success.
ALTERNATIVE DESIGNS
Once objectives are defined, alternative designs can be
considered. This is another time when the development
worker may want to seek some additional planning assistance.
For example, the problems of a particular area may
indicate the need for specialized expertise. In a case
where one of the alternative designs includes an irrigation
system, consultation with ecologists, irrigation
engineers, water resource managers or agricultural
economists
would be recommended before going very far with the
planning process.
Such consultations may involve local, national or
international
contacts. If local resource people are available,
an interdisciplinary team can be organized to visit the
possible project sites. The team can discuss the project
from their respective viewpoints. Depending on the type
of project, the team might include representatives from
several of these fields: ecology, hydrology, soil
science, geology and so on.
As planning continues locally, the development worker may
also want to get in touch with other organizations and
individuals around the world. See the list in Appendix
II.
Even when and if the project seems to be relatively simple
and easily tackled, it is a good idea to pass the proposed
alternatives through a review process and outside appraisal.
The information and data collected in the planning
process thus far will provide reviewers with excellent
background information to which they can respond.
COSTS/BENEFITS
In this manual, an analysis of the costs/benefits <see
sample> of a
eswx1320.gif (600x600)
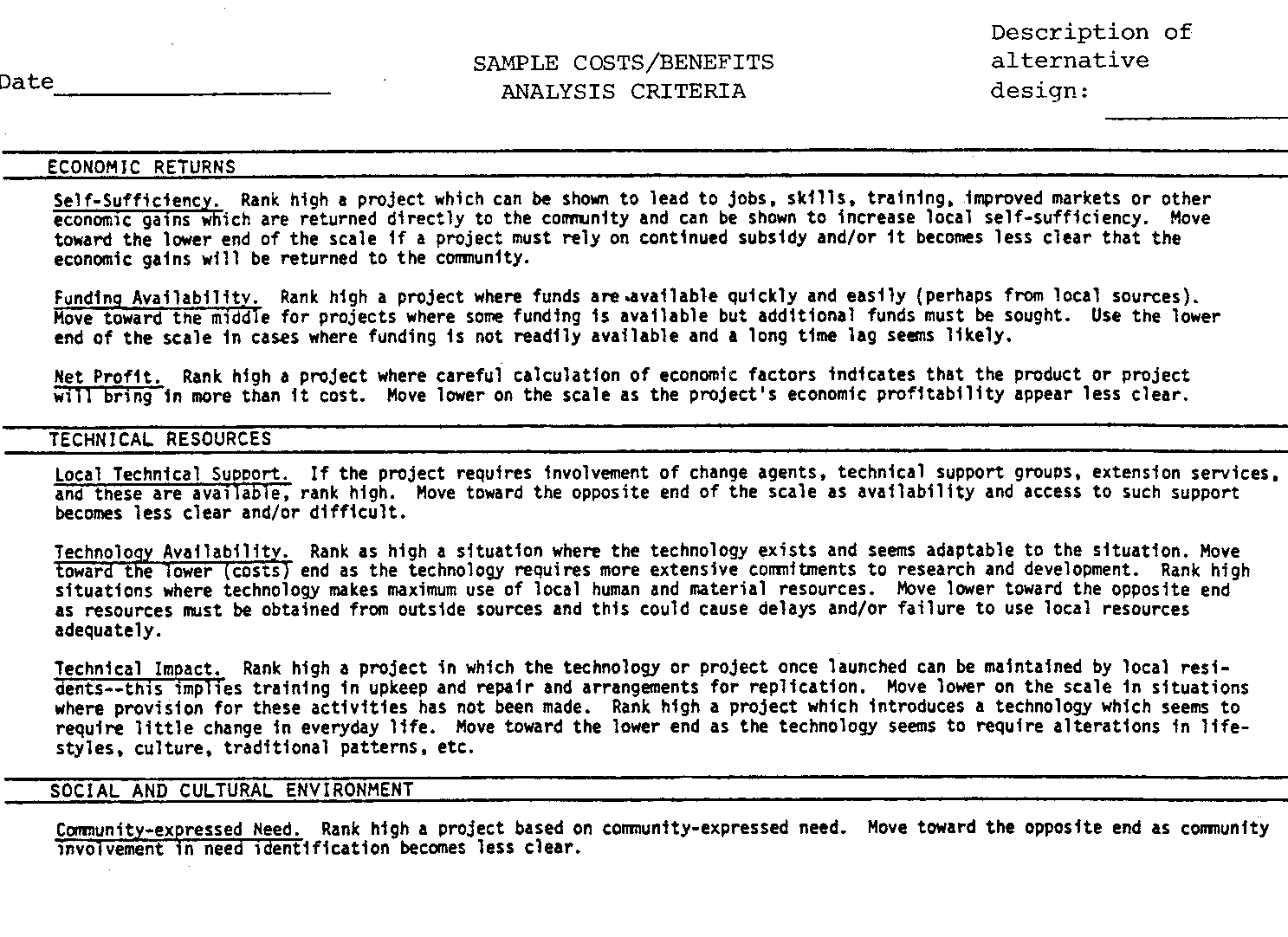
project is based on a comparison of the alternative
designs to four categories of criteria based on 1)
guidelines
set at the beginning of the planning process and 2)
the stated project objectives.
The guidelines, as discussed earlier, and the project
objectives cover many subjects. For purposes of analyzing
water projects, the following criteria are suggested:
*
economic returns
*
technical resources
*
social and cultural environment
*
physical environment.
For each of the criteria, some explanation is offered
here. However, the development worker will want to expand
this to be more specific.
The alternative designs are evaluated and measured for
each of the four criteria by using a simple scale
numbered from 1 to 10. The lower end (left) of the scale
represents costs or negative effects; the upper end
(right) represents benefits or positive effects. The five
point mark in the middle of the scale represents a
situation where benefits and costs are evenly balanced.
The four ratings are then averaged to give the development
worker a total average for the design. Alternative
designs can then be compared and the development worker
can select the design which appears most beneficial.
There is no magic about this measuring system; it seems
convenient and relatively easy to use. Development
workers are encouraged to adapt the system to fit a
particular situation.
IMPLEMENTATION OF PROJECT
Community participation should be factored into project
implementation as much as possible. For instance, if
villagers are willing to manually dig trenches for buried
pipe, this is preferable to the speedier method of using
heavy machinery. Projects should encourage the use of
local materials and local craftsmen wherever possible
instead of relying on imported materials or machinery. In
this way, future maintenance is not likely to be beyond
the local resources. Community pride developed through
community participation is the best guarantee for
continued maintenance and long-term benefits.
MONITORING
Small projects may not have small effects on the
environment;
the impacts of any project may be smaller or larger
than the scope of the project itself. Also, changes
caused by a water project may not be readily apparent,
perhaps being masked by the successful achievement of
project
objectives. No one can hope to predict all impacts
because the environmental interactions are very likely
more complex than we are able to comprehend. Therefore it
is important to continue to monitor the project after it
has been implemented.
A simple program of measuring change should be developed
before the project begins. This may consist of inspections
of water courses for the amount of sedimentation or
growth of waterweeds. Or it may require weekly or monthly
measurements of water levels in wells, ponds or other
water bodies. Even these simple measurements can identify
trends which may be harmful if allowed to persist. The
post-project monitoring can also help to identify maintenance
procedures necessary for continued operation.
Unforeseen benefits may be encouraged, while negative
trends may be corrected before the problems become too
severe. In water resource development, post-project
monitoring
and maintenance are as important as pre-project
planning.
APPENDIX I
BIBLIOGRAPHY OF CITED REFERENCES
Following is a selected list of publications for use in
small-scale planning. No doubt others of equal value are
available, but the information contained in these references
should provide enough information for any small-scale
water development task. Addresses of publishers or
distributors
of the references can be found in Appendix II.
Cairncross, S. and R. Feachem. 1978. Small Water
Supplies.
Bulletin No. 10, Ross Institute and
Advisory
Service, London.
Small, compact
reference with technical information,
diagrams and
designs for small-scale water
supply
projects.
Chakroff, M. 1976. Freshwater Fish Pond Culture and
Management.
VITA, Mt. Rainier, Maryland.
Lengthy
paperback on planning, construction and
operation of
fish culture ponds. Inexpensive.
Feachem, R., M. McGarry and D. Mara (eds.). 1977.
Water, Wastes
and Health in Hot Climates. John
Wiley &
Sons, New York.
An expensive,
scholarly collection of papers on
all aspects of
water and health in tropical
climates. Much
of the information is very useful
in the
planning and design of water projects. It
is difficult
to obtain, but it is a highly recommended
reference.
International Development Research Centre. 1978.
Appropriate
Sanitation Alternatives: A Field
Manual. Energy,
Water, and Telecommunication
Department,
Washington, D.C.
A clearly
illustrated manual of intermediate
technology.
Litzenberger, S.C. (ed.). 1974. Guide for Field Crops
in the Tropics
and Subtropics. Agency for
International
Development, Washington, D.C.
Exceptional
reference on the standard field crops
normally
cultivated in the tropics. Data on
cultivation,
requirements and productivity for
most tropical
crops.
Mann, H. T. and D. Williamson. 1976. Water Treatment
and Sanitation:
Simple Methods for Rural Areas.
Intermediate
Technology Publications, Ltd.,
London.
How-to
reference with readable text and excellent
diagrams.
McJunkin, F. E. 1975. Water, Engineers, Development and
Disease in the
Tropics. Agency for International
Development,
Washington, D.C.
A classic in
disease prevention design for
engineers and
project planners. While all
water-related
disease is covered, the focus is on
schistosomiasis.
Meynell, P. J. 1976. Methane: Planning a Digester.
Prism Press,
Dorset, U.K.
A small,
easily readable book on biogas
production.
Information is given on planning
design,
construction and operation, plus data on
productivity and
raw materials.
National Academy of Sciences. 1974. More Water for Arid
Lands: Promising
Technologies and Research
Opportunities.
National Academy of Sciences,
Washington, D.C.
Great
discussion on methods for conserving and
exploiting water sources in arid regions.
Many
of the
suggested methods can be applied to
small-scale
projects.
National Academy of Sciences. 1979. Tropical Legumes:
Resources for
the Future. National Academy of
Sciences,
Washington, D.C.
Good
discussion of many species of tropical
legumes not
traditionally cultivated throughout
the tropics
but which possess potential for
development.
National Academy of Sciences. 1975. Underexploited
Plants with
Promising Economic Value. National
Academy of
Sciences, Washington, D.C.
An exciting
book for small-scale planners.
Thirty-six
species of plants are described in
terms of
potential economic development.
Quigg, P. W. 1976. Water, the Essential Resource.
National Audubon
Society, International Series No.
2., New York.
A brief paper
that describes the global water
crisis. It
provides a proper perspective for the
small-scale
planner.
Rajagopalan, S. and M. Shiffman. 1974. Guide to Simple
Sanitary
Measures for the Control of Enteric
Diseases. World
Health Organization, Geneva.
An excellent
manual for the planning, design and
operation of
water supply and sanitary systems.
Ram, E. R. 1979. "Safe Water - Essential to
Health."
Contact 52,
August, 1979. World Council of
Churches,
Geneva.
A very handy
small publication covering the most
essential
aspects of safe water supplies.
Stern, P. H. 1979. Small Scale Irrigation.
Intermediate
Technology Ltd., London and the
International
Irrigation Information Center, Bet
Dagan, Israel.
The best
reference for small-scale irrigation,
includes
technical aspects, design and sound
advice.
United Nations. 1980. "UNICEF News" 103/1980/1.
Available from
UNICEF office or UNICEF National
Committee in
many countries.
A small,
regularly published pamphlet with
consistently
helpful information.
VITA. 1979. Environmentally Sound Small Scale
Agricultural
Projects. VITA-Mohonk Trust, Mt.
Rainier,
Maryland.
Similar to
this manual in format, providing
information
on planning and design of small-scale
agricultural
projects.
VITA. 1977. Village Technology Handbook. Mt. Rainier,
Maryland.
A
one-of-a-kind reference. Contains a treasury
of
information on a wide range of village level
projects. Has
been modified and reprinted
several
times, with input from field workers.
Wagner, E. G. and J. N. Lanoix. 1958. Excreta Disposal
for Rural Areas
and Small Communities. World Health
Organization,
Geneva.
The best
there is.
Wagner, E. G. and J. N. Lanoix. 1959. Water Supply for
Rural Areas and
Small Communities. World Health
Organization,
Geneva.
A
still-valuable classic on water supply. Much
of the
material in this book still serves as the
basis for
newer works.
Winblad, U. 1972. Evaluation of Waste Disposal Systems
for Urban, Low
Income Communities in Africa.
Scandinavian
Consulting Group for Planning,
Architecture and
Building, Copenhagen.
A small
reference which discusses the various
alternatives
of waste disposal. The discussion
and
recommendations are valid for rural areas.
The World Bank. 1976. Village Water Supply, A World
Bank Paper. The
World Bank, Washington, D.C.
World Neighbors. Contour Ditches Help Conserve Our Soil.
Volume 6, Number
2E. World Neighbors, Oklahoma
City, Oklahoma.
One of the
World Neighbors in Action' newsletters
which are
characterized by their simplicity
and
usefulness.
APPENDIX II
Where to Order References
(Complimentary copies of references are often sent to
people working in developing countries.)
Christian Medical Commission
World Council of Churches
150, Route de Ferney
1211 Geneva 20, Switzerland
Intermediate Development Technology Research Center
P.O. Box 8500
Ottawa, KIG 3H9, Canada
International Irrigation Information Center
Volcani Center
P.O. Box 49
Bet Dagan, Israel
or
International Irrigation Information Center
P.O. Box 8500
Ottawa, KIG 3H9, Canada
John Wiley and Sons, Inc.
605 Third Avenue
New York, New York 10016 USA
National Academy of Sciences
2101 Constitution Avenue, N.W.
Washington, D.C. 20418 USA
National Audubon Society
950 Third Avenue
New York, New York 10022 USA
Prism Press
Stable Court, Chalmington
Dorchester, Dorset, UK
Ross Institute of Tropical Medicine
London, UK
U.S. Agency for International Development
Washington, D.C. 20523 USA
VITA
1815 North Lynn Street, Suite 200
Arlington, VA 22209 USA
United Nations
New York, N.Y. 10017 USA
The World Bank
1818 H Street, N.W.
Washington, D.C. 20433 USA
World Health Organization
1211 Geneva 27
Switzerland
World Neighbors International Headquarters
5116 North Portland Avenue
Oklahoma City, Oklahoma 73112 USA
========================================
========================================