TECHNICAL PAPER #51
UNDERSTANDING PEDAL POWER
By
David Gordon Wilson
Technical Reviewers
John Furber
Lawrence M. Halls
Lauren Howard
Published By
VITA
1600 Wilson Boulevard, Suite 500
Arlington, Virginia 22209 USA
Tel: 703/276-1800 . Fax: 703/243-1865
Internet: pr-info@vita.org
Understanding Pedal Power
ISBN: 0-86619-268-9
[C]1986, Volunteers in Technical Assistance
PREFACE
This paper is one of a series published by Volunteers in
Technical
Assistance to provide an introduction to specific
state-of-the-art
technologies of interest to people in developing countries.
The papers are intended to be used as guidelines to help
people choose technologies that are suitable to their
situations.
They are not intended to provide construction or
implementation
details. People are
urged to contact VITA or a similar organization
for further information and technical assistance if they
find that a particular technology seems to meet their needs.
The papers in the series were written, reviewed, and
illustrated
almost entirely by VITA Volunteer technical experts on a
purely
voluntary basis.
Some 500 volunteers were involved in the production
of the first 100 titles issued, contributing approximately
5,000 hours of their time.
VITA staff included Betsy Eisendrath
as editor, Suzanne Brooks handling typesetting and layout,
and
Margaret Crouch as project manager.
The author of this paper, VITA Volunteer David Gordon
Wilson, is
a mechanical engineer at Massachusetts Institute of
Technology.
The reviewers are also VITA Volunteers.
John Furber is a consultant
in the fields of renewable energy, computers, and business
development. His
company, Starlight Energy Technology, is based
in California.
Lawrence M. Halls is a retired mechanical engineer
who designed farm machinery for Sperry-New Holland for 23
years. Lauren Howard
is a thinker, inventor, and bicycling advocate.
She lives in Charlottesville, Virginia.
VITA is a private, nonprofit organization that supports
people
working on technical problems in developing countries.
VITA offers
information and assistance aimed at helping individuals and
groups to select and implement technologies appropriate to
their
situations. VITA
maintains an international Inquiry Service, a
specialized documentation center, and a computerized roster
of
volunteer technical consultants; manages long-term field
projects;
and publishes a variety of technical manuals and papers.
UNDERSTANDING PEDAL POWER
by VITA Volunteer David Gordon Wilson
I. INTRODUCTION
Throughout history human, energy has generally been applied
through the use of the arms, hands, and back.
With minor
exceptions, it was only with the invention of the
sliding-seat
rowing shell, and particularly of the bicycle, that legs
also began to be considered as a "normal" means of
developing
power from human muscles.
A person can generate four times more power (1/4 horsepower
(hp)) by pedaling than by hand-cranking.
At the rate of
1/4hp, continuous pedaling can be done for only short
periods, about 10 minutes.
However,, pedaling at half this
power (1/8 hp) can be sustained for around 60 minutes.
Pedal
power enables a person to drive devices at the same rate as
that achieved by hand-cranking, but with far less effort and
fatigue. Pedal power
also lets one drive devices at a faster
rate than before (e.g. winnower), or operate devices that
require too much power for hand-cranking (e.g. thresher).
Over the centuries, the treadle has been the most common
method of using the legs to produce power.
Treadles are still
common in the low-power range, especially for sewing
machines.
Historically, two treadles were used for some
tasks, but even then the maximum output would have been
quite
small, perhaps only 0-15 percent of what an individual using
pedal operated cranks can produce under optimum conditions.
However, the combination of pedals and cranks, which today
seems an obvious way to produce power, was not used for that
purpose until quite recently.
It was almost 50 years after
Karl von Krais invented the steerable foot-propelled bicycle
in 1817 that Pierre Michaud added pedals and cranks, and
started the enormous wave of enthusiasm for bicycling that
has lasted to the present.
The main use of pedal power today is still for bicycling, at
least in the high-power range (75 watts and above of
mechanical power).
In the lower-power range there are a
number of uses of pedal power--for agriculture,
construction,
water pumping, and electrical generation--that seem to be
potentially advantageous, at least when electrical or
internal-combustion engine power is unavailable or very
expensive.
II. OPERATING PRINCIPLES
POWER LEVELS
The power levels that a human being can produce through
pedaling
depend on how strong the pedaler is and on how long he or
she
needs to pedal. If
the task to be powered will continue for
hours at a time, 75 watts mechanical power is generally
considered the limit for a larger, healthy non-athlete.
A healthy
athletic person of the same build might produce up to twice
this
amount. A person who
is smaller and less well nourished, but not
ill, would produce less; the estimate for such a person
should
Probably be 50 watts for the same kind of power production
over
an extended period.
The graph in Figure 1 shows various record
upp1x2.gif (600x600)
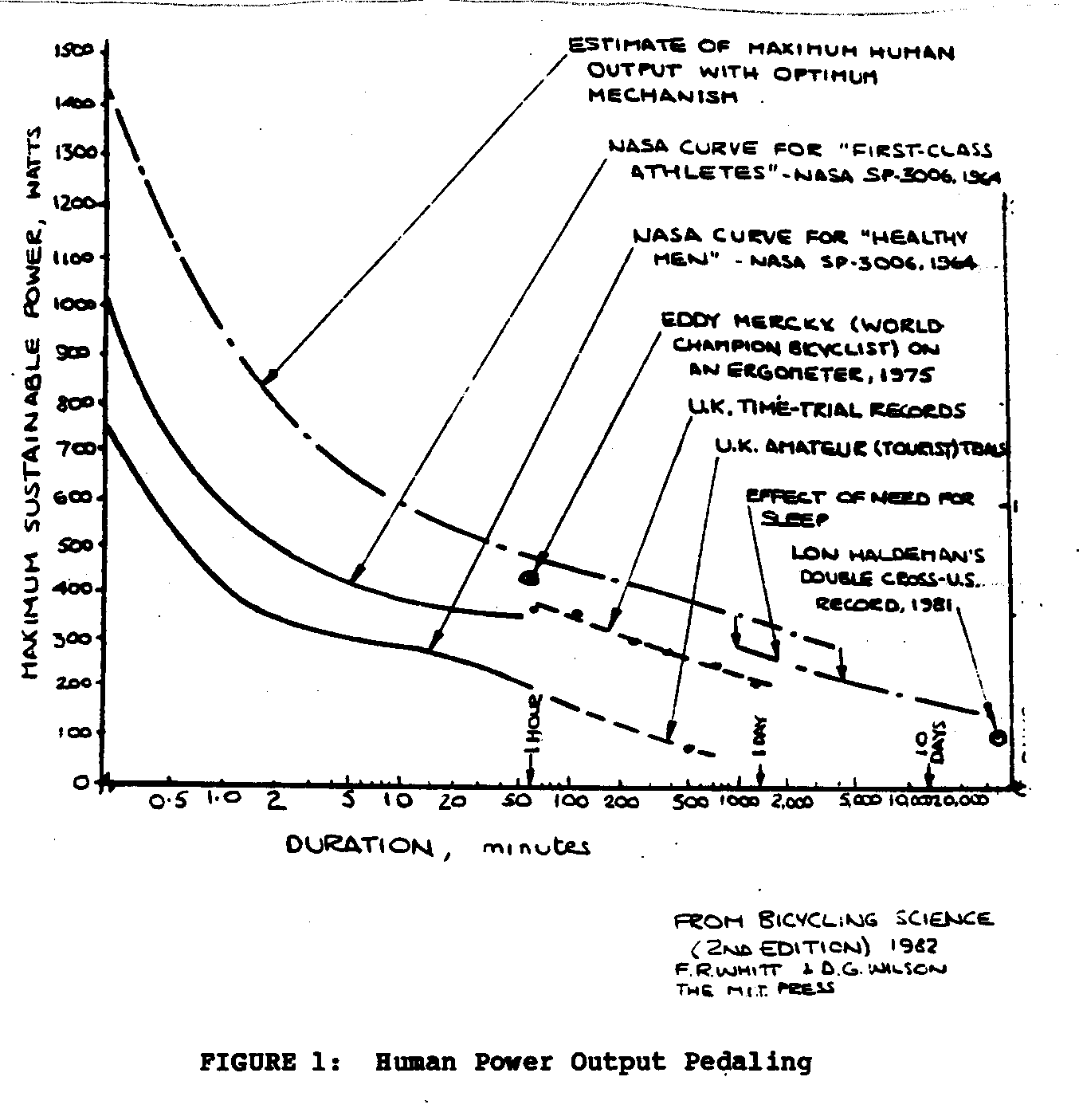
limits for pedaling under optimum conditions.
The meaning of
these curves is that any point on a curve indicates the
maximum
time that the appropriate class of person could maintain the
given average power level.
Power levels are also directly related to the environment of
the
person doing the pedaling.
To be able to continue pedaling over
an extended period, a person must be able to keep
cool--whether
because the ambient temperature is low enough, or because
there
is adequate breeze.
There is a vital difference between pedaling a stationary
device
and pedaling a bicycle at the same power output.
On a bicycle,
much of the pedaling energy goes into overcoming wind
resistance;
this wind resistance, however, provides an important
benefit:
cooling. Because of
the wind, even in hot, humid climates, so
long as the bicyclist drinks enough liquids, dehydration and
heat
stroke are unlikely to occur.
On the other hand, when pedaling a stationary device on a
hot or
humid day at more than about half the maximum possible power
output, there is a considerable danger of the pedaler's
collapsing
because of an excessive rise in body temperature.
Therefore,
it is essential that an individual pedaling such a
stationary
device in hot or humid conditions be provided with shade
from the sun, plenty of water, and preferably some sort of
fan.
A portion of the power that the pedaler is producing can be
used
to drive this fan; this is an efficient use for the power,
since
it will help prevent damage to the pedaler's health.
PEDALING RATE
How fast should a person pedal? Human beings are very
adaptable
and can produce power over a wide range of pedaling speeds.
However, people can produce more power--or the same amount
of
power for a longer time--if they pedal at a certain
rate. This
rate varies from person to person depending on their
physical
condition, but for each individual there is a pedaling speed
somewhere between straining and flailing that is the most
comfortable, and the most efficient in terms of power
production.
(For centuries, this fact was apparently not
recognized. The
predominant method of human power production was to strain
with
maximum strength against a slowly yielding resistance.
This is
neither comfortable nor efficient.
Neither is the opposite extreme
of flailing at full speed against a very small resistance.
A simple rule is that most people engaged in delivering
power
continuously for an hour or more will be most efficient when
Pedaling in the range of 50 to 70 revolutions per minute
(rpm).
See Figure 2. For
simplicity's sake, we will use 60 rpm, or one
upp2x4.gif (486x486)

revolution of the pedal cranks per second, as an easy
reference
value for estimates of the gear ratios required to drive a
given
load.
GEAR RATIOS
The relationship between the rotating speed of whatever is
being
driven and the pedaling rate (both expressed in revolutions
per
minute) is called the gear ratio.
Most practical applications of
Pedal power will use bicycle-chain drives, which on bicycles
range from 1:1 (the rear wheel turns at the same speed as
the
turns at five times the speed of the cranks) for high gears.
Very-Low-Power Applications
There are some very-low-power applications of pedal power,
in
which the required power output is so far below that of
which
human beings are capable that maximum efficiency is not a
concern.
For example, sewing machines are generally limited to a
less than optimum value to allow the sewing table to be
placed at
a convenient height.
The pedaler provides a range of sewing
speeds without gear-change mechanisms.
A large step-up ratio is
usually given by a round belt made of leather.
It cannot
transmit large torques; this inability serves a purpose,
because
when the sewing needle jams, the belt slips, preventing the
needle from breaking.
High-Power Applications
An example of an application at the higher-power end of the
scale
is a hypothetical maximum-power drive for an irrigation
pump.
Let us suppose that the pump has the speed-versus-power
characteristics
shown in Figure 3, and that the pedalers will be paid
upp3x5.gif (486x486)
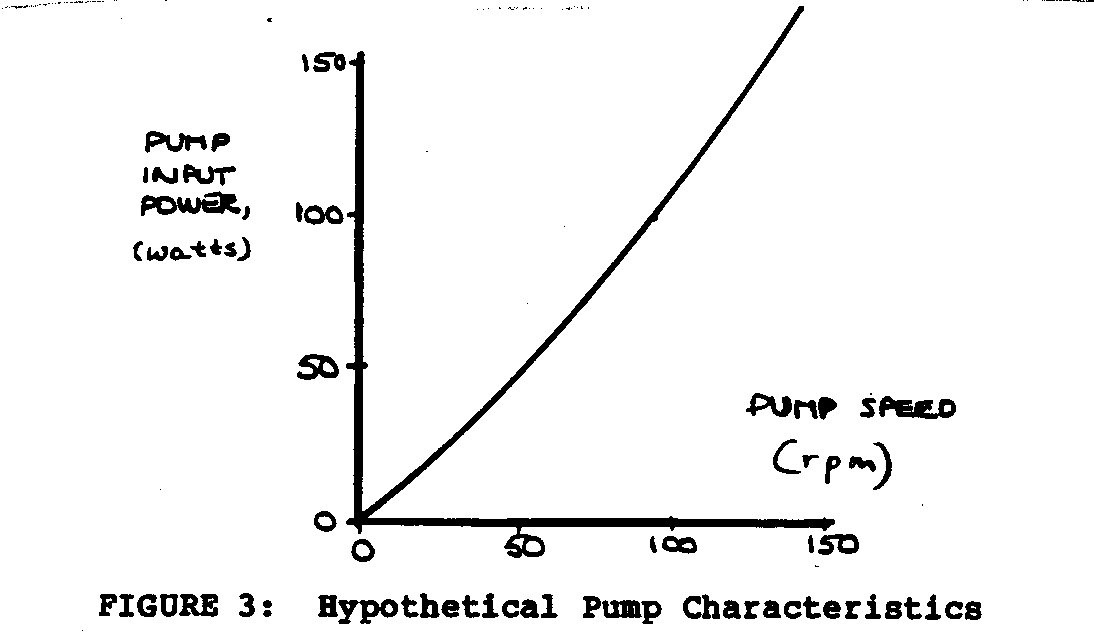
to produce as much power as they comfortably can for periods
of
two hours at a time.
Choosing a conservative value from Figure 1, we estimate
that a
mechanical output of 100 watts seems reasonable for this
length
of time.
Furthermore, we estimate from Figure 2 that the optimum
pedaling speed to give this power output is 55 rpm.
We can then
see from Figure 3 that when the pump absorbs 100 watts, its
speed
of revolution should be 95 rpm.
We need, therefore, a step-up
gear of 95:55. We
have available a set of bicycle cranks and
pedals with a chainwheel having 48 teeth.
To achieve our ratio
of 95:55, we then need
45 x 55/95 = 27.8
teeth on the cog (the smaller sprocket
attached to the
pump shaft).
Ideally then, we should use a sprocket of 28 teeth.
However,
sprockets of 27, 28, or 29 teeth would be acceptable.
No allowance has been made in this calculation for energy
losses
in the chain transmission.
This is because a single chain going
over two sprockets is very efficient--over 95 percent, even
for
unlubricated, worn, or dirty chains.
However, some applications require two stages of step-up
transmission,
and in these cases, power losses are greater.
For
instance, suppose that a ventilation fan must be driven for
a
long period at 900 rpm, and the optimum pedaling speed is
estimated to be 60 rpm.
The step-up ratio is then 900:60 = 15:1.
The smallest sprockets generally available for bicycles have
12
teeth. The
chainwheel for a single step-up stage would need:
12 x 15 = 180 teeth.
Such a chainwheel is not available, but even if one were
specially
made, it would have a diameter far too large to pedal
around.
Moreover, using a very large-chainwheel with a very small
cog
produces a small angle of contact (or wrap) around the cog;
this
causes high tooth wear on the cog.
Therefore, a step-up ratio of 15:1 is better produced by a
two-stage
step-up gear. For
example, a standard high-gear arrangement
from a bicycle could be used.
It has a chainwheel of 48
teeth driving a cog of 13 teeth, fixed to a second shaft on
its
own bearings(*) (for instance, another bicycle crankset with
another chainwheel of 48 teeth on the countershaft, driving
a cog
of 12 teeth on the shaft to be driven.
The combination would
then be
(48/13) x (48/12) = 14.8.
this is close enough to 15:1 to be useful.
In this case, it would be best to assume that there would be
a 10
percent loss of power.
For example, if the pedaler can produce
an output of 50 watts for the desired period, the driven
device
will receive 45 watts input.
III. DESIGN VARIATIONS
CRANK LENGTH
The crank length is the distance between the center of the
pedal-spindle
and the crank axis; that is, it is the radius of the
circle defined by each pedal as it turns.
The normal crank on an
adult's bicycle is 165 to 170 millimeters (mm) long.
However,
people remain able to produce near maximum power output at
any
crank length from between 165 and 180 mm, so long as they
have a
period to practice pedalling at the new length.
SHAPE OF CHAINWHEEL
Evidence from tests suggests that elliptical chainwheels
with a
relatively small degree of elongation--that is, with a ratio
of
major to minor axis of the chainwheel ellipse of no more
than
1.1:1--do allow most pedalers to produce a little more
power. No
subject tested showed a reduction in power.
It is therefore
recommended that, when elliptical chainwheels are available
at a
reasonable price, they be used.
However, the gain in power
output is small, and this must be considered when comparing
costs
and benefits.
PEDALING POSITIONS
There are three common pedaling positions:
o The
first is the upright position used by the majority
of cyclists around the world.
In this position, the seat, or
saddle, is located slightly behind where it would be if it
were a
seat, or vertically above the crank axis; the hand grips are
placed so that the rider leans forward just slightly when
pedaling. Tests have
shown that subjects using this position are
able to produce the most pedalling power when the top of the
saddle is fixed at a distance 1.1 times the leg length to
the
pedal spindle at the pedal's lowest point.
o
The second position is the position used by
riders of
racing bicycles with dropped handlebars, when they are
holding
the upper parts of the bars.
Their back is then at a forward
lean of about 40 degrees from the vertical.
Their saddle height
requirements are similar to those of cyclists in the first
position.
(The position of the racing bicyclist who is trying to
achieve maximum speed is not suitable for power production
on a
stationary device.
Even racing bicyclists sometimes experience
great pain after a long time in this position, and the
position
is unnecessary on a stationary device because there is no
wind
resistance to overcome.
o
The third position is the position used in
modern semi-recumbent
bicycles. The
placement of the center of the pedaling
circle relative to the seat is shown in Figure 4.
In this seating
upp4x7.gif (600x600)

position, the pedaling forces are countered by the lower
back
pushing into the seat (which is similar in construction to a
lawn
chair made of tubes and canvas).
The arms and hands do not need
to remain on the handlebars to perform this function, the
way
they usually do in the first two positions.
They can remain
relaxed, and free to guide the work that the pedaler is
powering.
The upper body too can remain relaxed, and the chest is in a
position that makes breathing easier than when the pedaler
bends
forward. The major
disadvantage of this position is that, since
the pedaler's legs move forward from the body, it may be
hard to
position large, deep equipment like a lathe or saw so that
it is
in reach without being in the way.
In almost all other respects,
the semi-recumbent position is highly desirable, though not
essential.
IV. APPLICATIONS OF PEDAL POWER
PEDAL POWER FOR TRANSPORTATION
The principal use of pedal power around the world is for the
transportation of people and goods.
A bicycle used by itself can
carry a rider, plus 50 to 100 kilograms of goods in a front
and/or rear carrier on the cross-bar, or on the rider's
head.
The carrying capacity of a bicycle can be greatly increased
by
attaching a trailer to it.
One model of trailer has seats for
two adults, and allows the bicycle to be easily converted
into a
rickshaw.
A rickshaw is usually made from the front or rear-portion of
a
standard bicycle, connected to a load-carrying platform over
a
two-wheel axle.
Rickshaws can carry an extraordinary quantity of
people and goods. In
Bangladesh, they are responsible for
transporting several times the total freight and passengers
carried
by all railroads, trucks, and buses combined.
However, the potential productivity of these rickshaws is
greatly
reduced by the fact that virtually every one of them has
only a
single-speed gear.
Sometimes the gear mechanism is even deliberately
converted to single speed.
When the author lived in
Nigeria, the most common bicycle was a Raleigh Roadster,
which
came equipped with a three-speed gear, but the retailers
normally
wired the gear-change lever in the low-gear position.
A driver
who has only one gear available must exert much more effort
to
pedal the rickshaw.
The strain is severe and unnecessary, and
productivity is greatly reduced.
OXFAM, an international development and relief organization,
has
done considerable work on a three-wheeled pedal operated
vehicle
capable of carrying payloads of over 150 kilograms.
Called an
"Oxtrike," the vehicle uses a three-speed gearbox
in its transmission
and a mild steel sheet frame.
The frame can be manufactured
on a small scale, using foot-powered cutters, hand
operated folding machines, and welding or riveting.
It can be
fitted with passenger seats or a cargo box.
It should be noted that an ordinary bicycle frame is not
sturdy
enough for payloads of this size.
The weight also requires
particularly good brakes.
The Oxtrike's mild steel construction
addresses the first problem.
The braking system includes both
the standard lever brakes on the front and a foot pedal on
the
rear. Each wheel has
its own brake drum, with a balance bar to
distribute the force equally.
OTHER USES OF PEDAL POWER
General Considerations
Broadly speaking, applications of pedal power are possible
when
the power level required is below a quarter of a horsepower
(that
is, below about 200 watts).
Common applications of stationary
pedal power include pumping water, grinding grains or
metals,
shredding, or threshing.
Pedal power can also be used to generate electricity for
individual
uses--to operate room lights, a television set, or a
projector, for example.
Surplus power could be diverted to a
battery-charging circuit.
The easiest way to do this is simply
to drive either a DC generator or an AC alternator through a
circuit feeding a battery in parallel with the load.
The same
circuit could be used for an alternator of higher power,
chain-driven
from the cranks, through an appropriate gear ratio.
Other pedal power applications include:
o
Cassava graters
o
Coffee pulpers
o
Coffee/grain hullers
o
Cracking of oil palm nuts
o
Fiber decorticaters--sisal, manila, hemp,
etc.
o
Winches or hoists
o
Balers
o
Potter's wheels
o
Flexible shaft drive for portable grinders,
saws, etc.
o
Tire pumps
o
Sewing machines
The use of pedal power for agricultural implements that have
to
move across soft or uneven ground is not recommended.
A mower
described and illustrated in Bicycling Science (see
Bibliography)
required so much energy to move the mower across the ground
that
a small proportion of the rider's total energy went into
mowing.
This loss of energy would occur for any use--like plowing,
cultivating,
or harrowing--in which the vehicle had to move across the
ground. McCullagh's
Pedal Power describes some designs in which
the pedaling unit is stationary at one edge of the plot,
while
the plow or other implement, guided by a second worker, is
pulled
by a rope or chain.
This principle should be tried out further.
The Dynapod
Bicycles can sometimes be adapted to drive the devices
mentioned
above, but the result is often inefficient.
It is frequently
cheaper in initial and maintenance costs to use a properly
designed and constructed dynapod.
A dynapod is a portable pedaling device that consists of a
stand,
saddle, handlebar, pedals, and sprocket wheel.
The name comes
from the Greek words for "power" and
"foot." Dynapod power
varies according to the size and fitness of the operator and
the
length of time spent pedaling.
There are three kinds of dynapods: 1) A one-person dynapod
that
utilizes belt drive.
It can be built either with or without
chaindrive. 2) A two-person dynapod that can be pedaled
either
by one person at a time, or by two people together.
It is also
possible to fit a special adaptor so that a direct shaft
drive
leads off the unit and powers a flour mill or other machine.
(When this is done, only one person can pedal at a time.) 3)
A
one-person dynapod that has belt drive, chain drive, and
direct
drive. It is very
similar to the two-person dynapod.
The two-person dynapod illustrated below (Figure 5) has been
upp5x10.gif (600x600)
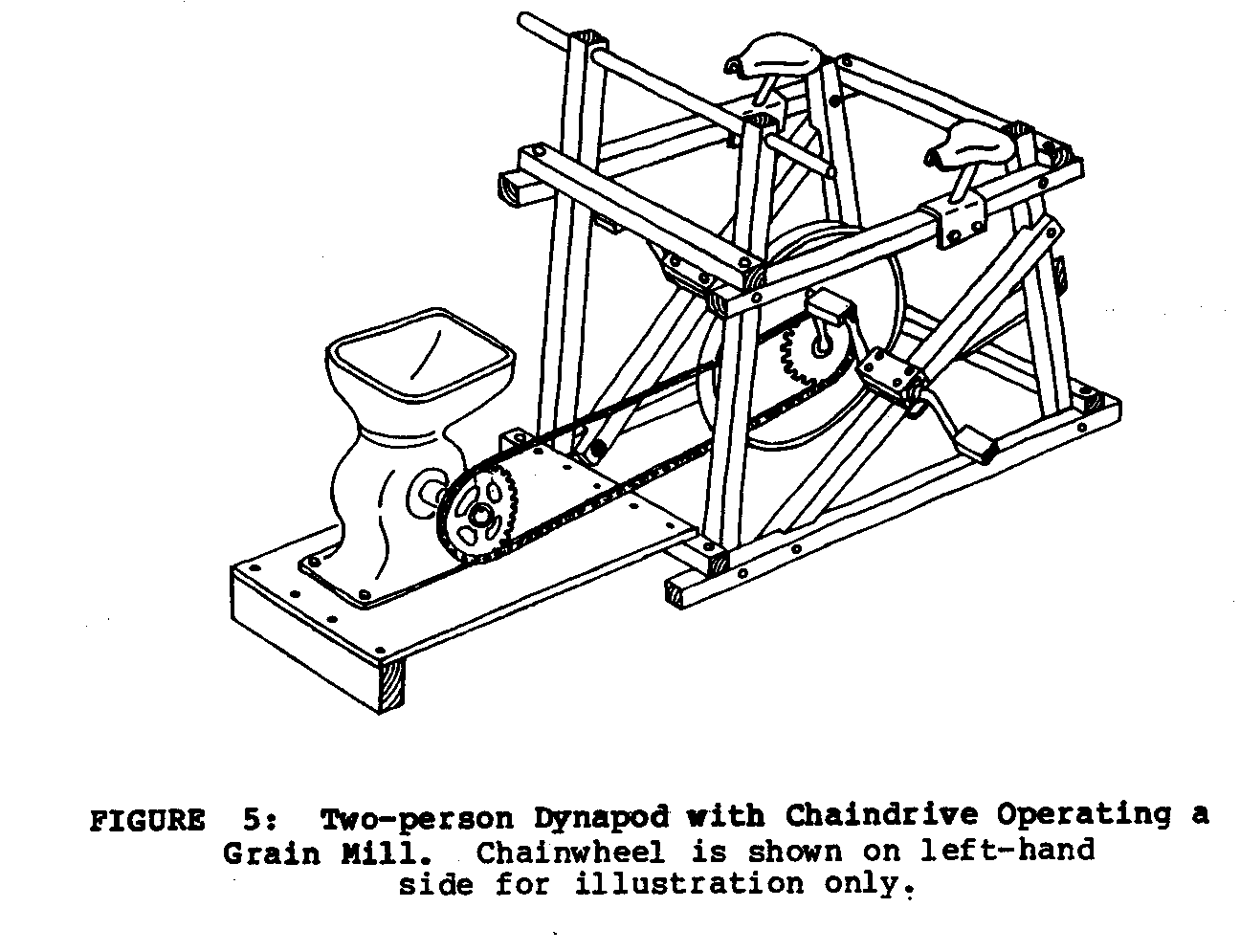
attached to a grain mill, but the unit can be adapted to a
wide
variety of uses. The
dynapod frame can be made of wood or welded
steel, depending on cost and availability of materials.
heavily weighted flywheel provides extra power and smoothes
out
the pedal stroke, reducing operator fatigue.
A similar device, designed to draw irrigation water from
shallow
wells in Bangladesh, consists of a welded steel frame with a
drive wheel attached to the plunger of a handpump (Figure
6).
upp6x11.gif (600x600)
V. COMPARING THE ALTERNATIVES AND CHOOSING THE APPROPRIATE
TECHNOLOGY
Whether or not pedal power is advantageous for an
application
depends absolutely on local conditions.
In many countries, gasoline
(petrol) is highly subsidized; one consequence is that
pedaled rickshaws, for example, are giving way to ones run
by
small internal combustion engines.
However, in locations far
from reliable fuel supplies and maintenance shops, internal
combustion engines are not so attractive an alternative, and
pedal-powered devices may be much more suitable.
Pedal power is often a comfortable choice because it is a
familiar technology--even though it may be used in
unfamiliar
ways. Construction
and maintenance skills are generally
available among village artisans, as are spare parts from
used
bicycles.
Depending on the application, pedal power can be extremely
versatile. A dynapod
can operate a pump during the growing
season, run a thresher at harvest time, and power a grain
mill
throughout the year.
Its portability allows it to be moved from
site to site to go where the work is.
Choice of pedal power--or any technology--must be made by
carefully comparing the alternatives for the application
desired
and the availability of resources--construction and
maintenance
skills, financing, raw materials, labor.
Whether machines are
made locally or purchased from nearby factories or even
importers, they should be within the capability of local
people
to maintain and repair in order to avoid so far as possible
lengthy periods when they are out of service.
At this level especially, pedal power often compares
favorably
with wind or water power.
A windmill, for example, often
requires considerably more resources in funds and
construction
time and skill than does a pedal-power unit based on a
bicycle.
Windmills within the capability of a local village builder
will
generally be limited to a size giving an average output of
50 to
100 watts (though the maximum output may be 1
kilowatt). Windmills
are more subject to the vagaries of the weather.
While
energy storage can help minimize the effect of variations in
the
wind, high winds and other kinds of severe weather often
cause
minor damage, and occasionally cause major damage.
Windmills in this range are probably best suited to charging
batteries
and to pumping water for irrigation, stock watering, or
potable water supply.
But even for these purposes, a pedaled
pump might be preferable.
It was found by the author's VITA
group, for instance, that the output of a Savonius-rotor
windmill
in average Boston area winds was so small--even when the
windmill
was mounted on a headland overlooking the sea--that the
windmill's
output could be exceeded by a pedal-power unit operated
for only two to four hours per week.
A pedaled pump may have several advantages if a farmer
wishes to
irrigate field crops: it is often less expensive to build;
it
can supply water at the most suitable times of the day and
of the
crop growth cycle; and it can be used at more than one
location
and possibly for more than one purpose.
BIBLIOGRAPHY
Darrow, Ken, and Pam, Rick.
"Energy: Pedal Power," from
Appropriate
Technology Sourcebook pp.189-196.
Stanford,
California:
Volunteers in Asia, Inc., 1977.
Kerr, Richard.
Rickshaw Study -- Bangladesh. Ottawa,
Canada:
Inter Pares,
1983. (Draft.)
McCullagh, James C., editor.
Pedal Power. Emmaus,
Pennsylvania:
Rodale Press, 1974.
"Pedal Power," a supplement to Energy for Rural
Development.
Washington, D.C.:
National Academy Press, 1981, pp. 137-148.
Weir, Alex.
Four-Person Pedal-Powered Grain Thresher/Mill.
Arlington,
Virginia: Volunteers in Technical Assistance (VITA),
1979.
Weir, Alex. The
Dynapod: A Pedal-Power Unit. Arlington,
Virginia:
Volunteers in Technical Assistance (VITA), 1980.
Whitt, Frank Rowland, and Wison, David Gordon.
Bicycling Science.
2nd ed. Cambridge,
Massachusetts: The MIT Press, 1983.
========================================
========================================